**In today’s society, electronic products are widely used in both work and daily life, making their reliability crucial. Most electronic systems and devices rely on printed circuit boards (PCBs), which must adhere to proper design principles to ensure optimal performance. Proper PCB design can significantly improve the reliability of these systems. For instance, if two thin parallel tracks are placed too close together, signal waveforms may be delayed, and excessive reflected noise can ultimately affect the terminal equipment.**
### 1. **Design Considerations for Grounding**
Many interference issues in electronic equipment can be resolved through correct shielding and proper grounding. Therefore, grounding design is a critical aspect of PCB design. A grounding system typically consists of four parts: analog ground, digital ground, chassis ground, and system ground. The digital ground is also called logical ground, while the chassis ground is often referred to as shield ground. Below are key points to consider in grounding design:
#### 1.1 **Selecting the Right Grounding Method**
There are generally two types of grounding methods: multi-point grounding and single-point grounding. The choice depends on the system’s operating frequency. If the equipment operates above 10 MHz, the impedance of a single ground wire may be too high, which could disrupt normal operation. In this case, multiple grounding points should be used to minimize ground impedance. Conversely, for circuits operating below 1 MHz, a single-point grounding method is more effective in preventing circulating currents that could cause interference. For circuits with operating frequencies between 1 and 10 MHz, multiple grounding points are recommended when the wavelength is within 20 times the length of the ground wire; otherwise, a single-point grounding method should be used.
#### 1.2 **Separating Analog and Digital Circuits**
PCBs are often complex, containing both analog and digital circuits. To avoid interference between the two, it is essential to separate them. One effective approach is to ground their power terminals separately. Additionally, the grounding area for analog circuits should be as large as possible to minimize noise coupling.
#### 1.3 **Choosing a Thicker Ground Wire**
If a ground wire is too thin, the resulting current fluctuations can alter the ground potential, leading to instability in the equipment and reduced noise immunity. To ensure stable operation and improve noise resistance, it is advisable to use thicker ground wires with higher current-carrying capacity. If possible, select wires that are 3 mm or wider.
### 2. **Design Considerations for Electromagnetic Compatibility (EMC)**
Given the complex and dynamic operating environments of electronic equipment, ensuring good electromagnetic compatibility (EMC) is essential. Proper EMC design helps reduce electromagnetic interference (EMI) to other devices and ensures the equipment’s ability to function in a variety of electromagnetic environments. The following points are crucial for improving EMC:
#### 2.1 **Choosing the Correct Routing Method**
In PCB layout, parallel routing can reduce the inductance of wires, but it may also increase distributed capacitance and mutual inductance between traces. When possible, a “tic-tac-toe” routing pattern can be used, where traces on one side of the board run vertically and those on the other side run horizontally, with vias connecting the traces at cross-points. This approach can minimize crosstalk between traces. Additionally, long-distance parallel routing should be avoided to reduce interference.
#### 2.2 **Selecting the Proper Wire Width**
To mitigate interference and control transient currents, it is essential to manage the inductance of the PCB traces. Inductance is inversely proportional to the width of the trace and proportional to its length. Therefore, shorter, thicker traces are more effective in suppressing interference. For high-current signals, such as those from bus drivers, clock leads, or row drivers, short traces should be used. For integrated circuits (ICs), the trace width should typically be between 0.2 mm and 1 mm, while for discrete component circuits, a width of around 1.5 mm is recommended.
### 3. **Design Considerations for Component Placement and PCB Dimensions**
The size of the PCB should be balanced. A board that is too large can result in long traces, which increase impedance, reduce noise resistance, and raise costs. In terms of component placement, devices that are functionally related should be positioned close together to improve noise immunity. For instance, clock generators, crystal oscillators, and CPU clock inputs, which are prone to noise, should be placed in close proximity. It is also important to separate noise-sensitive circuits, low-power components, and high-current components from the logic circuits to prevent interference. If possible, using separate PCBs for different sections is a good practice.
### 4. **Key Considerations for Heat Dissipation Design**
Effective heat dissipation is critical for maintaining the performance and longevity of electronic components. The PCB should ideally be installed in an upright position, with a gap of at least 2 cm between stacked boards. The arrangement of components should follow specific guidelines to optimize cooling:
– For systems that rely on natural convection cooling, integrated circuits (or other components) should be oriented vertically to facilitate air flow.
– For systems using forced air cooling, devices should be arranged horizontally to align with the airflow direction.
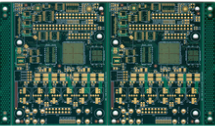
1. Devices on the same printed circuit board should, to the greatest extent possible, be arranged according to their heat output and thermal dissipation capabilities. Devices with low heat generation or poor heat resistance (such as small signal transistors, small-scale integrated circuits, electrolytic capacitors, etc.) should be placed at the top of the cooling airflow (at the intake). In contrast, devices that generate more heat or have higher thermal resistance (such as power transistors, large-scale integrated circuits, etc.) should be positioned at the furthest point downstream of the cooling airflow.
2. Horizontally, high-power devices should be placed as close to the edge of the board as possible to minimize the heat transfer path. Vertically, high-power components should be placed near the top of the board to minimize their effect on the temperature of surrounding devices.
3. Temperature-sensitive components should ideally be placed in the coolest areas of the board (such as near the bottom). They should never be positioned directly above heat-generating components. It is also preferable to stagger multiple devices on the horizontal plane to improve thermal distribution.
4. Heat dissipation in the printed circuit board relies primarily on airflow, so it is essential to consider the airflow path during the design phase. The placement of components and the layout of the PCB should be optimized accordingly. Air always tends to flow toward areas of lower resistance, so it is important to avoid leaving large empty spaces in specific areas when arranging devices on the board.
If your have any questions about PCB ,please contact me info@wellcircuits.com