In recent years, the emerging surface mount technology (SMT) has largely replaced traditional through-hole insertion methods, becoming the dominant force in the development of electronic equipment. SMT is widely regarded as a revolutionary advancement in electronic assembly technology. Its primary objectives are to enhance product reliability and performance while reducing costs. This technology is set to significantly transform both consumer and military electronic products alike.
2. Introduction to Surface Mount Technology and PCB Components
Surface mount technology (SMT) is an electronic assembly method where components are directly mounted onto the surface of a printed circuit board (PCB), without the need for drilled insertion holes. Solder is used to create both the mechanical and electrical connections between the components and the PCB.
Electronic products utilizing SMT typically consist of printed circuit boards and surface mount components. A printed wire board (PWB) is a single-sided or multilayer material that includes both conductive traces and pads. Surface mount components can be divided into two categories: surface mount passive components and surface mount devices. Passive components include various types of resistors, capacitors, and inductors, while surface mount devices (SMDs) refer to packaged active components, such as small outline packages (SOP), ball grid arrays (BGA), and others. However, not all components are suitable for SMT; for example, certain connectors, transformers, and large capacitors may not be used in SMT-based designs.

**3. Surface Mount Technology Process**
Surface mount technology (SMT) involves two primary processes: the main process and the auxiliary process. The main process includes printing, component placement, and reflow soldering. All products, regardless of type, must undergo these three steps, with each part being crucial to the overall process. The auxiliary process mainly consists of the “dispensing” process and optical-assisted automatic inspection. While not always necessary, these auxiliary steps depend on the product characteristics and user requirements.
Printed circuit boards (PCBs) can be categorized into single-sided and double-sided products. Likewise, electronic products can also be classified as single-sided (components are placed on only one side of the PCB) or double-sided (components are placed on both sides). Figure 1 illustrates the SMT process flow for single-sided products, while Figure 2 shows the process flow for double-sided products.
The purpose of the printing process is to apply solder paste accurately to the PCB through the coordinated actions of a stencil and printing equipment. Key elements in this process include the solder paste, stencil, and the printing system. Solder paste plays a vital role in establishing both electrical and mechanical connections between the components and the PCB. Composed primarily of an alloy and flux, solder paste performs different functions during the welding process to ensure proper soldering. The stencil is used to apply solder paste precisely onto the PCB. The design and manufacturing of the stencil significantly impact print quality. The printing system refers to the equipment and the printing parameters involved. The quality of printing equipment greatly affects printing accuracy. Achieving accurate printing depends on the optimal combination of the printing equipment’s repeatability and the correct settings for printing parameters. Key parameters influencing print quality include printing speed, squeegee pressure, demolding speed, and demolding distance. These parameters must be carefully set and matched to optimize printing quality. The printing speed typically ranges from 12.7 to 203.2 mm/s, depending on the squeegee pressure and the physical properties of the solder paste. The SMT process generally requires a squeegee pressure range of 4.448 to 6.672 N.
The purpose of the placement process is to ensure accurate and rapid attachment of components to the PCB. The key factor in this process is the placement machine’s capability. The placement accuracy of the machine is essential for proper component alignment. Key technologies in placement machines include motion control, execution, high-speed feeding mechanisms, miniaturization technologies, high-speed machine vision, intelligent control technologies, real-time multitasking, and flexible modular design for equipment and system integration.
The reflow soldering process involves melting the pre-applied solder paste on the PCB pads to create mechanical and electrical connections between the component leads and the PCB pads. Reflow soldering ensures reliable connections. The main elements of the reflow soldering process are the reflow oven and its soldering capabilities, which are influenced by the heating system, cooling system, flux management system, and inert gas protection system. The heating system’s efficiency, temperature control precision, uniformity, and stability play key roles in achieving optimal soldering. The cooling system is critical because rapid cooling prevents PCB warping caused by excessive heat. Additionally, it refines the solder structure and minimizes the growth of intermetallic compounds, improving reliability. During reflow soldering, flux volatilizes. If there is no proper flux management system to capture and filter the volatilized flux, it can condense in the cooling area, contaminating both the equipment and the PCB. In cases where solder paste activity is insufficient, or when dealing with fine-pitched or complex components, the reflow oven can be filled with inert gas (typically nitrogen) to minimize oxidation and improve soldering activity. The reflow oven’s performance is also controlled through its software, which governs the temperature stages. Typically, the PCB will pass through the preheating, soaking, reflow, and cooling stages in the reflow oven to ensure high-quality solder joints.
The auxiliary processes help support smooth assembly and aid in both proactive and post-assembly inspections. These processes are primarily the “dispensing” and optical-assisted automatic inspection. The dispensing process involves applying special glue to the underside or perimeter of the components to protect them. This ensures the components stay in place during reflow soldering and are shielded from environmental stresses that could cause them to detach or degrade. Key elements in dispensing include the dispensing equipment, special glue, and parameter settings, which must be optimized for the process. Optical-assisted automatic inspection involves using specialized optical equipment to measure solder paste thickness and printing accuracy after the paste is applied, verify placement accuracy after component placement, and detect circuit board defects before reflow soldering. After reflow, the equipment inspects solder joints and flags any defects. Common optical inspection tools include automated optical inspection (AOI) systems for visual solder joints and X-ray equipment, which can inspect non-visible joints, such as those under BGAs. The decision to employ these auxiliary processes depends on the specific requirements of the product being assembled.
**4. Principles and Temperature Curve of Reflow Soldering**
Reflow soldering operates based on a specific temperature curve (Fig. 3). When the PCB enters the preheating zone, the solvent and gas in the solder paste evaporate, and the flux begins to activate, cleaning the surfaces and moistening the pads and component pins. The solder paste softens, collapses, and covers the pads, isolating them from oxygen. As the PCB moves into the soaking zone, both the PCB and components are fully preheated, preventing thermal shock that could damage the board. Once the PCB reaches the reflow zone, the temperature rises rapidly to melt the solder paste, enabling the solder to wet and bond the pads, component leads, and pins. Finally, in the cooling zone, the solder solidifies, completing the reflow process.
The reflow soldering process involves multiple phases, each with specific temperature ranges. The temperature zones are divided as follows: the preheating zone is from room temperature to 120°C, the soaking zone is from 120°C to 170°C, the reflow zone spans from 170°C to 230°C, and the cooling zone cools from approximately 210°C to 100°C.
The temperature curve is critical for ensuring soldering quality. The heating rate and peak temperature should be aligned with the temperature profile of the solder paste. The ramp-up rate before 160°C should be controlled at 1–2°C/s. Rapid heating can lead to component or PCB damage due to excessive temperature changes, or it can cause the solvent in the solder paste to evaporate too quickly, resulting in solder ball formation. The peak temperature is typically set 20–40°C higher than the solder paste’s melting point (e.g., for Sn63Pb37 solder paste with a melting point of 183°C, the peak should be set at 205°C–230°C), with the time above the liquidus being 10–60 seconds. A low peak temperature or insufficient reflow time can result in poor solder joints, while a high peak temperature or prolonged reflow time can lead to oxidation of the solder paste, compromising solder joint quality.
The reflow soldering temperature profile is primarily determined by factors such as solder paste specifications, PCB material, board thickness, component size and density, and the type of components used, including BGAs or CSPs. Equipment factors such as oven length, heating source material, and reflow furnace construction also influence the temperature curve settings.
In actual production, a reflow oven may have several temperature zones: heating, soaking, rapid heating, and reflow zones. The solder paste used is typically Sn63Pb37, with a melting point of 183°C. Specific reflow soldering parameters, such as temperature ramp rates and dwell times in each zone, are critical for achieving a proper reflow profile. Figure 4 shows the standard reflow soldering temperature curve, while Figure 5 presents the actual temperature curve for a specific PCB.
A reflow oven with nine temperature zones is used, with three test points for measuring actual temperatures. The key parameters for temperature zone settings include: 1) ramp-up rate to 100°C not exceeding 2°C/s; 2) soaking zone: 100°C–150°C for 70–120 seconds; 3) rapid heating zone: 150°C–183°C for a maximum of 30 seconds, heating rate of 2–3°C/s; 4) reflow zone: 205°C–230°C, with 40–60 seconds above liquidus; 5) cooling zone: cooling rate of 2–4°C/s. By comparing the theoretical and actual PCB temperature curves in Figures 4 and 5, it is evident that the actual temperature profile stays within the standard range, ensuring that the reflow soldering meets quality standards and ensures the electrical performance of the mounted components. Regular weekly checks of the reflow oven, including comparisons of the test temperature curve with the standard profile, are essential to maintain consistent soldering quality.
**5. Conclusion**
Surface mount technology has become pervasive across many industries, directly influencing the soldering quality, performance, and reliability of electronic products. This paper discusses the entire SMT process, detailing the principles and temperature curve of reflow soldering. By comparing the standard reflow soldering temperature curve with the actual curve during production, it is clear that as long as the actual reflow temperature range aligns with the standard curve, the performance of the mounted components will meet the required specifications.
2. Introduction to Surface Mount Technology and PCB Components
Surface mount technology (SMT) is an electronic assembly method where components are directly mounted onto the surface of a printed circuit board (PCB), without the need for drilled insertion holes. Solder is used to create both the mechanical and electrical connections between the components and the PCB.
Electronic products utilizing SMT typically consist of printed circuit boards and surface mount components. A printed wire board (PWB) is a single-sided or multilayer material that includes both conductive traces and pads. Surface mount components can be divided into two categories: surface mount passive components and surface mount devices. Passive components include various types of resistors, capacitors, and inductors, while surface mount devices (SMDs) refer to packaged active components, such as small outline packages (SOP), ball grid arrays (BGA), and others. However, not all components are suitable for SMT; for example, certain connectors, transformers, and large capacitors may not be used in SMT-based designs.
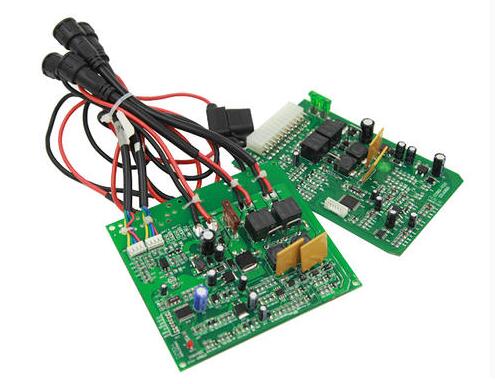
**3. Surface Mount Technology Process**
Surface mount technology (SMT) involves two primary processes: the main process and the auxiliary process. The main process includes printing, component placement, and reflow soldering. All products, regardless of type, must undergo these three steps, with each part being crucial to the overall process. The auxiliary process mainly consists of the “dispensing” process and optical-assisted automatic inspection. While not always necessary, these auxiliary steps depend on the product characteristics and user requirements.
Printed circuit boards (PCBs) can be categorized into single-sided and double-sided products. Likewise, electronic products can also be classified as single-sided (components are placed on only one side of the PCB) or double-sided (components are placed on both sides). Figure 1 illustrates the SMT process flow for single-sided products, while Figure 2 shows the process flow for double-sided products.
The purpose of the printing process is to apply solder paste accurately to the PCB through the coordinated actions of a stencil and printing equipment. Key elements in this process include the solder paste, stencil, and the printing system. Solder paste plays a vital role in establishing both electrical and mechanical connections between the components and the PCB. Composed primarily of an alloy and flux, solder paste performs different functions during the welding process to ensure proper soldering. The stencil is used to apply solder paste precisely onto the PCB. The design and manufacturing of the stencil significantly impact print quality. The printing system refers to the equipment and the printing parameters involved. The quality of printing equipment greatly affects printing accuracy. Achieving accurate printing depends on the optimal combination of the printing equipment’s repeatability and the correct settings for printing parameters. Key parameters influencing print quality include printing speed, squeegee pressure, demolding speed, and demolding distance. These parameters must be carefully set and matched to optimize printing quality. The printing speed typically ranges from 12.7 to 203.2 mm/s, depending on the squeegee pressure and the physical properties of the solder paste. The SMT process generally requires a squeegee pressure range of 4.448 to 6.672 N.
The purpose of the placement process is to ensure accurate and rapid attachment of components to the PCB. The key factor in this process is the placement machine’s capability. The placement accuracy of the machine is essential for proper component alignment. Key technologies in placement machines include motion control, execution, high-speed feeding mechanisms, miniaturization technologies, high-speed machine vision, intelligent control technologies, real-time multitasking, and flexible modular design for equipment and system integration.
The reflow soldering process involves melting the pre-applied solder paste on the PCB pads to create mechanical and electrical connections between the component leads and the PCB pads. Reflow soldering ensures reliable connections. The main elements of the reflow soldering process are the reflow oven and its soldering capabilities, which are influenced by the heating system, cooling system, flux management system, and inert gas protection system. The heating system’s efficiency, temperature control precision, uniformity, and stability play key roles in achieving optimal soldering. The cooling system is critical because rapid cooling prevents PCB warping caused by excessive heat. Additionally, it refines the solder structure and minimizes the growth of intermetallic compounds, improving reliability. During reflow soldering, flux volatilizes. If there is no proper flux management system to capture and filter the volatilized flux, it can condense in the cooling area, contaminating both the equipment and the PCB. In cases where solder paste activity is insufficient, or when dealing with fine-pitched or complex components, the reflow oven can be filled with inert gas (typically nitrogen) to minimize oxidation and improve soldering activity. The reflow oven’s performance is also controlled through its software, which governs the temperature stages. Typically, the PCB will pass through the preheating, soaking, reflow, and cooling stages in the reflow oven to ensure high-quality solder joints.
The auxiliary processes help support smooth assembly and aid in both proactive and post-assembly inspections. These processes are primarily the “dispensing” and optical-assisted automatic inspection. The dispensing process involves applying special glue to the underside or perimeter of the components to protect them. This ensures the components stay in place during reflow soldering and are shielded from environmental stresses that could cause them to detach or degrade. Key elements in dispensing include the dispensing equipment, special glue, and parameter settings, which must be optimized for the process. Optical-assisted automatic inspection involves using specialized optical equipment to measure solder paste thickness and printing accuracy after the paste is applied, verify placement accuracy after component placement, and detect circuit board defects before reflow soldering. After reflow, the equipment inspects solder joints and flags any defects. Common optical inspection tools include automated optical inspection (AOI) systems for visual solder joints and X-ray equipment, which can inspect non-visible joints, such as those under BGAs. The decision to employ these auxiliary processes depends on the specific requirements of the product being assembled.
**4. Principles and Temperature Curve of Reflow Soldering**
Reflow soldering operates based on a specific temperature curve (Fig. 3). When the PCB enters the preheating zone, the solvent and gas in the solder paste evaporate, and the flux begins to activate, cleaning the surfaces and moistening the pads and component pins. The solder paste softens, collapses, and covers the pads, isolating them from oxygen. As the PCB moves into the soaking zone, both the PCB and components are fully preheated, preventing thermal shock that could damage the board. Once the PCB reaches the reflow zone, the temperature rises rapidly to melt the solder paste, enabling the solder to wet and bond the pads, component leads, and pins. Finally, in the cooling zone, the solder solidifies, completing the reflow process.
The reflow soldering process involves multiple phases, each with specific temperature ranges. The temperature zones are divided as follows: the preheating zone is from room temperature to 120°C, the soaking zone is from 120°C to 170°C, the reflow zone spans from 170°C to 230°C, and the cooling zone cools from approximately 210°C to 100°C.
The temperature curve is critical for ensuring soldering quality. The heating rate and peak temperature should be aligned with the temperature profile of the solder paste. The ramp-up rate before 160°C should be controlled at 1–2°C/s. Rapid heating can lead to component or PCB damage due to excessive temperature changes, or it can cause the solvent in the solder paste to evaporate too quickly, resulting in solder ball formation. The peak temperature is typically set 20–40°C higher than the solder paste’s melting point (e.g., for Sn63Pb37 solder paste with a melting point of 183°C, the peak should be set at 205°C–230°C), with the time above the liquidus being 10–60 seconds. A low peak temperature or insufficient reflow time can result in poor solder joints, while a high peak temperature or prolonged reflow time can lead to oxidation of the solder paste, compromising solder joint quality.
The reflow soldering temperature profile is primarily determined by factors such as solder paste specifications, PCB material, board thickness, component size and density, and the type of components used, including BGAs or CSPs. Equipment factors such as oven length, heating source material, and reflow furnace construction also influence the temperature curve settings.
In actual production, a reflow oven may have several temperature zones: heating, soaking, rapid heating, and reflow zones. The solder paste used is typically Sn63Pb37, with a melting point of 183°C. Specific reflow soldering parameters, such as temperature ramp rates and dwell times in each zone, are critical for achieving a proper reflow profile. Figure 4 shows the standard reflow soldering temperature curve, while Figure 5 presents the actual temperature curve for a specific PCB.
A reflow oven with nine temperature zones is used, with three test points for measuring actual temperatures. The key parameters for temperature zone settings include: 1) ramp-up rate to 100°C not exceeding 2°C/s; 2) soaking zone: 100°C–150°C for 70–120 seconds; 3) rapid heating zone: 150°C–183°C for a maximum of 30 seconds, heating rate of 2–3°C/s; 4) reflow zone: 205°C–230°C, with 40–60 seconds above liquidus; 5) cooling zone: cooling rate of 2–4°C/s. By comparing the theoretical and actual PCB temperature curves in Figures 4 and 5, it is evident that the actual temperature profile stays within the standard range, ensuring that the reflow soldering meets quality standards and ensures the electrical performance of the mounted components. Regular weekly checks of the reflow oven, including comparisons of the test temperature curve with the standard profile, are essential to maintain consistent soldering quality.
**5. Conclusion**
Surface mount technology has become pervasive across many industries, directly influencing the soldering quality, performance, and reliability of electronic products. This paper discusses the entire SMT process, detailing the principles and temperature curve of reflow soldering. By comparing the standard reflow soldering temperature curve with the actual curve during production, it is clear that as long as the actual reflow temperature range aligns with the standard curve, the performance of the mounted components will meet the required specifications.