1. What causes crosstalk?
When a signal travels along the PCB traces, its electromagnetic waves also extend along the traces, moving from one end of the integrated circuit chip to the other. During this propagation, electromagnetic waves induce transient voltages and currents due to electromagnetic induction. Electromagnetic waves consist of electric and magnetic fields that fluctuate over time. On a PCB, the electromagnetic field is not confined solely to the traces; a significant portion of the electromagnetic field energy exists outside these traces. Therefore, if there are adjacent lines, the electric and magnetic fields produced by a signal traveling along one trace can influence nearby traces. According to Maxwell’s equations, time-varying electric and magnetic fields induce voltages and currents in adjacent conductors. Consequently, the electromagnetic field accompanying the signal’s propagation causes signals to be generated in neighboring lines, resulting in crosstalk.
2. Capacitance characteristics of forward crosstalk
Forward crosstalk exhibits two interrelated characteristics: capacitive and perceptual. As the “intruding” signal advances, it generates a voltage signal with the same phase in the “victim” trace. This induced signal travels at the same speed as the “intruding” signal but appears slightly earlier. Thus, the crosstalk signal does not propagate ahead of the intruding signal; instead, it couples with more energy while maintaining the same speed. Since the variation in the “intruding” signal generates the crosstalk signal, the forward crosstalk pulse is not unipolar; it has both positive and negative polarities. The duration of the pulse corresponds to the switching time of the “intruding” signal.
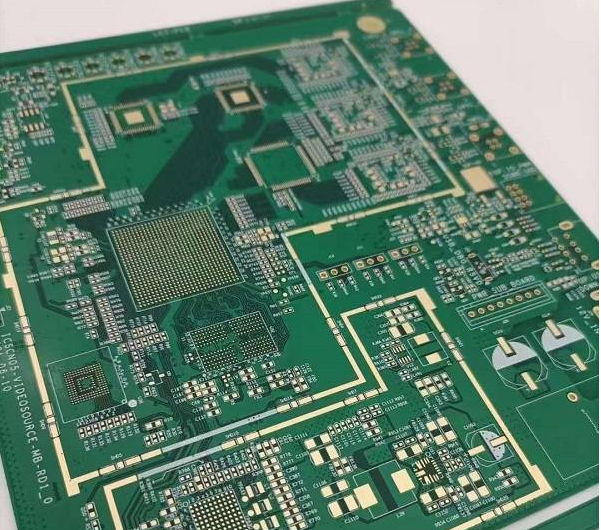
1. The coupling capacitance between wires dictates the amplitude of the forward crosstalk pulse, which is influenced by various factors including PCB material, geometric dimensions, and the positioning of crossing lines. The amplitude correlates with the distance between parallel lines: greater distances lead to larger crosstalk pulses. However, there is an upper limit to the crosstalk pulse amplitude, as the “intruding” signal progressively loses energy, while the “victim” line can couple back to the “intruder.”
2. Inductive characteristics of forward crosstalk come into play when the “intrusion” signal propagates, generating a time-varying magnetic field that causes crosstalk. Forward crosstalk, which exhibits inductive characteristics, differs significantly from capacitive crosstalk: their polarities are opposite. This is due to the competing effects of capacitive and inductive components in the forward direction, which can cancel each other out. In fact, when capacitive and inductive crosstalk are equal, no forward crosstalk occurs.
3. In many devices, forward crosstalk is relatively minor, while backward crosstalk can become a significant issue, particularly in long strip circuit boards, where capacitive coupling is heightened. Without simulation, it’s challenging to ascertain how much the inductive and capacitive crosstalk cancel each other.
4. If you measure forward crosstalk, you can discern whether your trace experiences capacitive or inductive coupling based on polarity. If the crosstalk polarity matches the “intrusion” signal, capacitive coupling prevails; otherwise, inductive coupling dominates. Typically, inductive coupling is stronger in PCBs.
5. The physical principles governing backward crosstalk mirror those of forward crosstalk: the time-varying electric and magnetic fields of the “intrusion” signal induce capacitive and inductive signals in the “victim.” However, there are notable differences.
6. The primary distinction lies in the duration of the backward crosstalk signal. As forward crosstalk and the “intrusion” signal propagate in the same direction and speed, their durations coincide. Conversely, backward crosstalk travels in the opposite direction, trailing the “intrusion” signal and creating an extended series of pulses.
7. Unlike forward crosstalk, the amplitude of backward crosstalk pulses does not depend on line length; its pulse duration is double the delay time of the “intrusion” signal. Why is this the case? When observing backward crosstalk from the signal’s origin, the “intrusion” signal, while distant, still generates backward pulses until another delayed signal appears. Consequently, the total duration of the backward crosstalk pulse is twice the delay time of the “intrusion” signal.
8. You might overlook the crosstalk interference between the driver chip and the receiver chip, but backward pulses warrant attention. Typically, driver chips have low-impedance outputs, which reflect more crosstalk signals than they absorb. When the backward crosstalk signal reaches the “victim’s” driver chip, it reflects back to the receiving chip. Since the output resistance of the driver chip is usually lower than that of the trace itself, this reflection often occurs.
9. Unlike forward crosstalk, which has both inductive and capacitive characteristics, backward crosstalk possesses only one polarity, preventing it from canceling itself out. The polarity of the backward crosstalk signal and the reflected crosstalk signal aligns with that of the “intrusion” signal, and its amplitude is the sum of both components.
10. Keep in mind that when measuring the backward crosstalk pulse at the “victim’s” receiving end, this crosstalk signal has already been reflected by the “victim” driver chip. You will observe that the polarity of the backward crosstalk signal is opposite to that of the “intrusion” signal.
11. In digital design, quantitative metrics are often essential. Regardless of when or how crosstalk occurs, whether forward or backward, the maximum noise tolerance is 150mV. So, is there a straightforward way to accurately measure noise? Unfortunately, the answer is “no,” as the electromagnetic field effects are complex, involving numerous equations, the PCB topology, chip analog characteristics, and more.
12. One approach to mitigate crosstalk is to modify one or more geometric parameters affecting coupling, such as trace length, distance between traces, and layering position on the PCB. Another strategy is to utilize terminals to convert single lines into multi-channel coupled lines. With thoughtful design, multi-line terminals can cancel a significant amount of crosstalk.
13. Many designers believe that reducing line length is crucial for minimizing crosstalk. However, nearly all circuit design software offers maximum parallel line length controls. Unfortunately, simply altering geometric values can be insufficient for reducing crosstalk.
14. Since forward crosstalk is influenced by coupling length, shortening lines with no coupling relationship results in minimal reduction of crosstalk. Moreover, if the coupling length exceeds the rise or fall time delay of the driver chip, the linear relationship between coupling length and forward crosstalk reaches saturation. At this point, shortening already lengthy coupling lines has little effect on crosstalk reduction.
15. Increasing the distance between coupled lines is not straightforward. In densely packed layouts, significant effort is needed to reduce wiring density. If crosstalk interference is a concern, consider adding one or two isolation layers. If you need to expand the distance between lines or networks, an easy-to-use software tool would be beneficial. While the width and thickness of traces also influence crosstalk interference, their impact is considerably less than that of distance. Therefore, these parameters are rarely adjusted.
16. The thickness of the dielectric material significantly affects crosstalk interference over large lengths. Generally, positioning the wiring layer close to the power layer (Vcc or ground) can help mitigate crosstalk interference. The precise improvement achieved should be determined through simulation.
17. Some PCB designers fail to prioritize layering methods, which is a critical error in high-speed circuit design. Layering influences transmission line performance, including impedance, delay, and coupling, and can lead to operational malfunctions or changes. For instance, simply reducing dielectric thickness by 5mil does not effectively mitigate crosstalk interference, even if it may be viable from a cost and processing perspective.
18. Unfortunately, such terminals are costly and ideal performance is challenging to achieve, as low coupling impedance between certain transmission lines can result in substantial current flow into the driver chip. The impedance between the transmission line and ground must not be excessively high for the driver chip to function effectively. If these issues arise and you plan to utilize this type of terminal, consider adding a few AC coupling capacitors.
19. Despite some implementation challenges, impedance array terminals remain a powerful solution for addressing signal reflection and crosstalk, especially under harsh conditions. In other environments, their effectiveness may vary, but they are still recommended.