2. Ensuring high reliability, defined as a product’s capability to meet all customer requirements within its expected lifespan, is paramount.
3. The reliance on electronic systems is escalating due to advancements in big data and artificial intelligence, heightening the consequences of reliability shortcomings.
4. At DesignCon 2020, I had the privilege to delve into how ANSYS empowers engineers to craft highly reliable products.
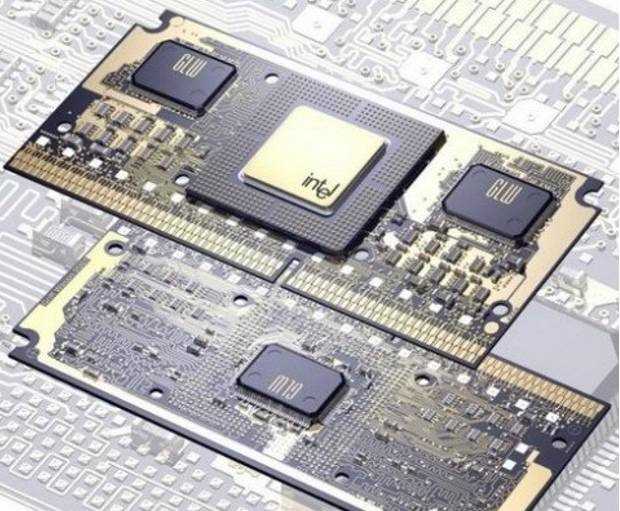
ANSYS, headquartered near Pittsburgh, Pennsylvania, was established in 1970 and currently boasts a team of approximately 4,000 experts specializing in finite element analysis, computational fluid dynamics, electronics, semiconductors, embedded software, and design optimization. Renowned for its expertise, ANSYS serves discerning clients in the aerospace and aviation sectors. Its expansion trajectory accelerated with strategic acquisitions within the EDA realm. In 2008, Ansoft Corp. was incorporated into its portfolio, followed by the integration of Apache Design Solutions in 2011. The acquisition spree continued in 2019 with ANSYS absorbing DfR Solutions, the sole provider of automated design reliability analysis software, Sherlock.
The amalgamation of Sherlock’s meticulous reliability analysis with ANSYS’s comprehensive multi-physics solutions yields a robust designer suite, facilitating swift and thorough electronic failure analysis early in the design phase. This synergy translates to considerable time and cost savings for users during product development. The addition of DfR electronic reliability solutions bolsters ANSYS’s prowess in semiconductor packaging, PCBA simulation, library generation, component analysis, and testing.
Traditional reliability analysis methods, involving prototype or pre-production unit testing, entail significant expenses and time investment, with results only emerging at the tail end of the product lifecycle. ANSYS Sherlock Finite Element Analysis (FEA) empowers engineers to assess hardware reliability seamlessly at the onset of the design process. This capability empowers designers to evaluate various architectures, geometries, and materials comprehensively, optimizing outcomes across diverse conditions.
In a packed conference venue, Kelly Morgan, ANSYS’s chief application engineer, showcased three instances where Sherlock could deliver substantial value by leveraging physical failure principles to forecast hardware reliability. The following is just a glimpse of the extensive information available:
Dielectric materials boasting a low dielectric constant (K) offer advantages such as reduced parasitic capacitance, enhanced circuit performance, and decreased power consumption. However, the diminished mechanical strength resulting from differences in thermal expansion coefficient (CTE) can occasionally precipitate cracks in the dielectric during reflows or thermal cycles. These cracks can be identified through acoustic examination. Discovery of cracks in low-K materials post-product launch necessitates a redesign cycle. Contrastingly, Sherlock and ANSYS empower IC designers to anticipate such failures at project inception, enabling prompt corrective measures to avert them.
1. Many integrated circuits traditionally utilize lead-free solder bumps as connections to other cores, packages, and even printed circuit boards (PCBs).
2. Variations in thermal expansion coefficients and temperatures in adjacent layers can result in differential expansion and contraction of materials.
3. These thermomechanical forces, along with vibrations, mechanical shocks, etc., can strain the solder joints, potentially leading to cracks in the solder joints and interconnect surfaces.
4. More recently, copper columns have gained popularity due to their smaller solder joint spacing.
5. However, these interconnections are more rigid and may be prone to quicker failure under applied strain.
6. The multi-physical features of Sherlock and ANSYS Mechanical enable users to easily and accurately predict the reliability of these interconnections, facilitating early adjustments to the design if necessary.
7. With the diminishing space in electronic devices, the utilization of micro-through hole technology in PCBs has surged.
8. Stacking micropores up to three or four stories high has become commonplace.
9. However, improper material selection and geometry in these designs may lead to unexpected cracking and layering in the micropores.
10. Thermal-mechanical stress, moisture, vibration, and other factors can result in the separation of micropores and layering of copper trace lines at the top or bottom of electroplated through holes (PTH).
11. Sherlock analyzes these problematic areas, considering overstress conditions during backflow and/or operation, and can forecast when fatigue may cause interconnect failures between through-holes, routing layers, and UBM contacts under convex points.
Even a point-of-best tool like Sherlock needs to be integrated into a user-friendly, highly productive design flow to provide its full value in a customer’s design environment. By utilizing upstream and downstream tools for smooth data exchange, engineers can swiftly and efficiently leverage Sherlock’s myriad capabilities. This integration of design flow minimizes scripting, data format conversion, and the error-prone, time-consuming manual intervention. Sherlock seamlessly interacts with ANSYS’s Icepak and ANSYS Mechanical, amalgamating these tools into a highly productive and dependable design process to achieve the “zero defect” goal demanded by a growing array of applications.
ANSYS Icepak furnishes robust electronic cooling solutions for thermal and fluid flow analysis of integrated circuits (ICs), packages, printed circuit boards (PCBs), and electronic components, employing the industry-leading ANSYS Fluent Computational Fluid Dynamics (CFD) solver. The ANSYS Icepak CFD solver employs the ANSYS Electronics Desktop (AEDT) graphical user interface (GUI).
This presents engineers with a CAD-centric solution, enabling them to tackle thermal challenges within the same unified framework as ANSYS HFSS, Ansys Maxwell, and Ansys Q3D Extractor, utilizing an easy-to-navigate functional area interface. Electrical and mechanical engineers operating in this environment benefit from a fully automated design process facilitating seamless coupling of HFSS, Maxwell, and Q3D Extractor to Icepak for steady or transient thermal analysis.
Engineers can depend on Icepak to provide integrated electronic cooling solutions for various electronic applications, from single ICs to packages, PCBs, computer casings, and entire data centers. The Icepak solver conducts conduction, convection, and radiation conjugate heat transfer analysis, equipped with advanced features to simulate laminar and turbulent flow, alongside multiple analysis types including radiation and convection. Icepak boasts an extensive library of fans, radiators, and materials offering solutions to commonplace electronic cooling challenges.
The imminent technology revolution of 5G connectivity is poised to reshape industries, ushering in a ubiquitous, ultra-fast computing network connecting billions of data-driven devices. This transformation will fuel economic expansion, spawn new products and services, and redefine our way of life. However, before 5G can fully deliver on its promise and meet quality-of-service (QoS) targets, wireless system designers and engineers must surmount numerous challenges. The Ansys 5G simulation solution equips stakeholders to streamline the complexity of device, network, and data center design.
The Ansys 5G Simulation solution encompasses electromagnetic, semiconductor, and electronic cooling and structural analysis tools to precisely simulate 5G radio and related technologies. Leveraging high-performance computing, this multi-solution platform can be deployed across enterprises, fostering more efficient collaboration between designers and engineering experts.
The phased-array design process in ANSYS HFSS entails a meticulous series of steps. ANSYS HFSS, a 3D high-frequency electromagnetic (EM) tool, is adept at designing and simulating a myriad of HF electronic products including antennas, antenna arrays, RF and microwave components, resonators, filters, and other HF electronic components. The phased array design process in HFSS commences with a single unit prototype, wherein antenna design parameters are optimized through the experimental design (DoE) method. Subsequently, the entire array is synthesized for simulation optimization of its overall performance in ANSYS HFSS, followed by modeling and simulation of the installed antenna and its interaction with the environment using a hybrid ANSYS HFSS SBR+ ray-tracing solver.