1. In recent years, foreign research on high-speed A/D converters has been particularly active, with notable advancements seen in the basic Flash structure [2]. These advancements include subranging circuit structures such as the half-flash, pipelined, multistage, and multistep structures. Essentially, these are circuit configurations comprising multiple Flash circuit structures and other functional circuits in various combinations. This approach addresses the limitations of the basic Flash circuit structure, resulting in high-speed, high-resolution A/D converters.
2. This type of structure is gradually supplanting the traditional SAR and integral structures. Another variation is the bit-per-stage circuit structure, which builds upon these improvements. One notable advancement is the Folding (or Mag Amps) structure, which features a Gray code serial output. These PCB circuit design techniques represent significant progress towards developing high-speed, high-resolution, and high-performance A/D converters, playing a crucial role in their advancement.

1. In addition, in the circuit design technology of high-resolution A/D converters, the Σ-Δ circuit structure is currently a widely adopted design technique.
2. This circuit structure is utilized not only in high-resolution low-speed or medium-speed A/D converters but is expected to gradually replace SAR and integral circuit structures.
3. When combined with the pipeline architecture, it is anticipated to achieve greater resolution and faster performance in A/D converters.
4. The PCB proofing clock duty cycle stabilization circuit plays a vital role amidst the continuous expansion and performance enhancements of electronic systems in the new era of military equipment.
5. This growth has led to increased complexity in electronic systems.
6. To ensure adequate capabilities and performance in data sampling, control feedback, and digital processing, modern military electronic systems are demanding higher specifications for A/D converters.
7. This is particularly true for military data communication systems and data acquisition systems, where the need for high-speed and high-resolution A/D converters continues to escalate.
8. The clock duty cycle stabilization circuit serves as the core unit of high-speed, high-precision A/D converters, significantly influencing the converter’s signal-to-noise ratio (SNR) and effective number of bits (ENOB).
9. Therefore, ensuring the performance of high-speed, high-precision A/D converters necessitates that the sampling and encoding clocks possess suitable duty cycles and minimal jitter.
10. Consequently, researching the clock duty cycle stabilization circuit becomes essential.
11. As the core unit of high-speed, high-precision A/D converters, the clock duty cycle stabilization circuit is rarely available as a standalone product, primarily documented in high-performance A/D converters.
12. In comparison to competing products, ADI’s offerings enhance sampling performance significantly due to advancements in the DCS (Duty Cycle Stabilizer) circuit.
13. The DCS circuit’s role is to minimize clock signal jitter, with sampling timing being dependent on these clock signals.
14. Previous DCS circuits from various companies have managed to control jitter to approximately 0.25 ps, whereas the new high-performance products like the AD9446 and LTC2208 reduce jitter to around 50 fs.
15. Generally, reducing jitter has the effect of improving SNR, thus increasing effective resolution (ENOB); this can achieve sampling rates exceeding 100 Msps while maintaining a 16-bit quantization level.
16. If the sampling rate increases without appropriate jitter control, ENOB will decrease, and the required resolution cannot be achieved.
17. Therefore, increasing the number of quantization bits becomes unfeasible.
18. With the development of high-performance A/D converters, DCS circuits are evolving towards higher speeds, reduced jitter, and enhanced stability.
19. Table 1 presents the clock duty cycle stabilization circuit technical parameters in foreign A/D converters.
20. To date, AD’s reported 60 fs jitter remains the lowest in the industry.
21. Typical aperture jitter is usually maintained around 1 ps, and jitter exceeding this value, even reaching tens of ps, proves to be of minimal significance.
22. The realization method for the PCB proofing clock stabilization circuit primarily involves using phase-locked loops (Phase-Locked Loop, PLL).
23. Essentially, the phase-locked system constitutes a closed-loop phase control structure.
24. In simple terms, it is a circuit capable of synchronizing the output signal with the input signal concerning both frequency and phase.
25. Therefore, once the system attains a locked state (or synchronized state), the phase difference between the oscillator’s output and the input signal is either zero or remains constant.
26. Owing to numerous advantageous characteristics, the phase-locked loop finds extensive applications in generating and distributing high-performance processor clocks, system frequency synthesis and conversion, automated frequency tuning and tracking, bit synchronization extraction in digital communication, and phase-locking operations.
27. This article proposes a delay-locked loop (DLL) design.
28. The PLL primarily employs a phase detector and filter to compare the feedback clock signal with the input clock signal, subsequently using the generated voltage difference to adjust the voltage-controlled oscillator and produce a signal similar to the input clock, thereby achieving frequency locking.
29. The DLL, on the other hand, introduces a delay pulse between the input clock and the feedback clock until the rising edges of both clocks align.
30. Once synchronization is achieved, and the edges of the input clock pulse and feedback pulse coincide, the on-chip delay phase-locked loop DLL can fully lock.
31. After locking the clock, the circuit remains stable, and the difference between the two clocks diminishes.
32. This mechanism allows the on-chip delay phase-locked loop to use the DLL output clock to compensate for time delays introduced by the clock distribution network, effectively enhancing the clock source and load.
33. First, the delay line introduces less noise compared to the oscillator.
34. This phenomenon occurs because any damaged zero-crossing points in the waveform disappear at the end of the delay line and are recycled within the oscillator circuit, generating more noise.
35. Furthermore, the delay time varies rapidly with changes in control voltage within the DLL, meaning that the transfer function simplifies to the gain KBCDL of the VCDL.
36. In summary, the oscillator utilized in the PLL may introduce instability and phase offset accumulation, which can diminish PLL performance due to time delays caused by network compensation clocks.
37. Therefore, the stability and stable speed of the DLL outperform the PLL significantly.
38. The PCB board test system integrates a novel PCB design approach, employing a USB bus-based automatic test system paired with virtual instrument design concepts.
39. This methodology maximizes the role of computers while replacing traditional instrument paradigms, thereby reducing instrument size and development costs, and increasing efficiency.
40. Following D/A conversion, the test system applies the required analog excitation signal, subsequently routing it to the switch matrix via the test bus.
41. The switch matrix, controlled by a microprocessor, manages the activation and deactivation of the test PCB board, which is secured on the needle bed.
42. The excitation signal is applied precisely to designated positions on the printed circuit board, allowing the test circuit to measure response.
43. The collected analog data is then relayed to the core control unit, where A/D conversion occurs, and the corresponding digital signals are processed by the software on the PCB machine to determine PCB board quality.
2. This type of structure is gradually supplanting the traditional SAR and integral structures. Another variation is the bit-per-stage circuit structure, which builds upon these improvements. One notable advancement is the Folding (or Mag Amps) structure, which features a Gray code serial output. These PCB circuit design techniques represent significant progress towards developing high-speed, high-resolution, and high-performance A/D converters, playing a crucial role in their advancement.
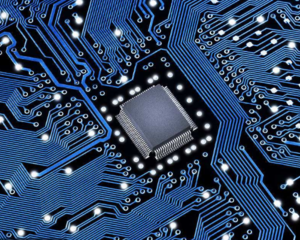
1. In addition, in the circuit design technology of high-resolution A/D converters, the Σ-Δ circuit structure is currently a widely adopted design technique.
2. This circuit structure is utilized not only in high-resolution low-speed or medium-speed A/D converters but is expected to gradually replace SAR and integral circuit structures.
3. When combined with the pipeline architecture, it is anticipated to achieve greater resolution and faster performance in A/D converters.
4. The PCB proofing clock duty cycle stabilization circuit plays a vital role amidst the continuous expansion and performance enhancements of electronic systems in the new era of military equipment.
5. This growth has led to increased complexity in electronic systems.
6. To ensure adequate capabilities and performance in data sampling, control feedback, and digital processing, modern military electronic systems are demanding higher specifications for A/D converters.
7. This is particularly true for military data communication systems and data acquisition systems, where the need for high-speed and high-resolution A/D converters continues to escalate.
8. The clock duty cycle stabilization circuit serves as the core unit of high-speed, high-precision A/D converters, significantly influencing the converter’s signal-to-noise ratio (SNR) and effective number of bits (ENOB).
9. Therefore, ensuring the performance of high-speed, high-precision A/D converters necessitates that the sampling and encoding clocks possess suitable duty cycles and minimal jitter.
10. Consequently, researching the clock duty cycle stabilization circuit becomes essential.
11. As the core unit of high-speed, high-precision A/D converters, the clock duty cycle stabilization circuit is rarely available as a standalone product, primarily documented in high-performance A/D converters.
12. In comparison to competing products, ADI’s offerings enhance sampling performance significantly due to advancements in the DCS (Duty Cycle Stabilizer) circuit.
13. The DCS circuit’s role is to minimize clock signal jitter, with sampling timing being dependent on these clock signals.
14. Previous DCS circuits from various companies have managed to control jitter to approximately 0.25 ps, whereas the new high-performance products like the AD9446 and LTC2208 reduce jitter to around 50 fs.
15. Generally, reducing jitter has the effect of improving SNR, thus increasing effective resolution (ENOB); this can achieve sampling rates exceeding 100 Msps while maintaining a 16-bit quantization level.
16. If the sampling rate increases without appropriate jitter control, ENOB will decrease, and the required resolution cannot be achieved.
17. Therefore, increasing the number of quantization bits becomes unfeasible.
18. With the development of high-performance A/D converters, DCS circuits are evolving towards higher speeds, reduced jitter, and enhanced stability.
19. Table 1 presents the clock duty cycle stabilization circuit technical parameters in foreign A/D converters.
20. To date, AD’s reported 60 fs jitter remains the lowest in the industry.
21. Typical aperture jitter is usually maintained around 1 ps, and jitter exceeding this value, even reaching tens of ps, proves to be of minimal significance.
22. The realization method for the PCB proofing clock stabilization circuit primarily involves using phase-locked loops (Phase-Locked Loop, PLL).
23. Essentially, the phase-locked system constitutes a closed-loop phase control structure.
24. In simple terms, it is a circuit capable of synchronizing the output signal with the input signal concerning both frequency and phase.
25. Therefore, once the system attains a locked state (or synchronized state), the phase difference between the oscillator’s output and the input signal is either zero or remains constant.
26. Owing to numerous advantageous characteristics, the phase-locked loop finds extensive applications in generating and distributing high-performance processor clocks, system frequency synthesis and conversion, automated frequency tuning and tracking, bit synchronization extraction in digital communication, and phase-locking operations.
27. This article proposes a delay-locked loop (DLL) design.
28. The PLL primarily employs a phase detector and filter to compare the feedback clock signal with the input clock signal, subsequently using the generated voltage difference to adjust the voltage-controlled oscillator and produce a signal similar to the input clock, thereby achieving frequency locking.
29. The DLL, on the other hand, introduces a delay pulse between the input clock and the feedback clock until the rising edges of both clocks align.
30. Once synchronization is achieved, and the edges of the input clock pulse and feedback pulse coincide, the on-chip delay phase-locked loop DLL can fully lock.
31. After locking the clock, the circuit remains stable, and the difference between the two clocks diminishes.
32. This mechanism allows the on-chip delay phase-locked loop to use the DLL output clock to compensate for time delays introduced by the clock distribution network, effectively enhancing the clock source and load.
33. First, the delay line introduces less noise compared to the oscillator.
34. This phenomenon occurs because any damaged zero-crossing points in the waveform disappear at the end of the delay line and are recycled within the oscillator circuit, generating more noise.
35. Furthermore, the delay time varies rapidly with changes in control voltage within the DLL, meaning that the transfer function simplifies to the gain KBCDL of the VCDL.
36. In summary, the oscillator utilized in the PLL may introduce instability and phase offset accumulation, which can diminish PLL performance due to time delays caused by network compensation clocks.
37. Therefore, the stability and stable speed of the DLL outperform the PLL significantly.
38. The PCB board test system integrates a novel PCB design approach, employing a USB bus-based automatic test system paired with virtual instrument design concepts.
39. This methodology maximizes the role of computers while replacing traditional instrument paradigms, thereby reducing instrument size and development costs, and increasing efficiency.
40. Following D/A conversion, the test system applies the required analog excitation signal, subsequently routing it to the switch matrix via the test bus.
41. The switch matrix, controlled by a microprocessor, manages the activation and deactivation of the test PCB board, which is secured on the needle bed.
42. The excitation signal is applied precisely to designated positions on the printed circuit board, allowing the test circuit to measure response.
43. The collected analog data is then relayed to the core control unit, where A/D conversion occurs, and the corresponding digital signals are processed by the software on the PCB machine to determine PCB board quality.