1. With the vigorous development of surface mount technology, the future trend of PCB (printed circuit boards) will inevitably move toward high-density packaging featuring fine lines, small holes, and multiple layers.
2. However, the copper plating process required for manufacturing such advanced circuit boards will encounter technical bottlenecks.
3. In recent years, the rapid advancement of the semiconductor and computer industries has made PCB production increasingly complex.
4. The complexity of a circuit board can be determined by the formula: PCB complexity index = (number of layers * number of wires between two solder joints) / (solder joint spacing in inches * wire width in mils).
5. For example, a 16-layer board with a solder joint pitch of 0.1 inches and a wire width of 5 mils, where there are three wires between the two solder joints, has a complexity index of 96.
6. Since the 1980s, the rise of surface mount technology has elevated the circuit board industry to new heights.
7. The advancement of multi-layer boards has led to a rapid increase in complexity indices, from around 20 in traditional circuit boards to 100 or higher in modern designs.
8. As technology and products evolve, encountering technical bottlenecks is inevitable.
9. Using the copper plating process as a case study, this article explores its fundamental principles and seeks solutions from three perspectives: macro, micro, and microstructure.

1. The macro aspect refers to the board surface of the PCB circuit board. Typically, the size of a large board is about 24″ x 18″. Achieving uniform coating thickness across the center and edges is challenging. According to Faraday’s law of electrolysis, the coating thickness is proportional to the applied current. Assuming a constant coating density, the thickness distribution mirrors the cathode current distribution.
2. Many factors affecting current distribution include the solution resistance, electrode polarization, plating geometry, and the distance between poles, as well as the magnitude of the applied current and the mass transfer rate. We will delve into these effects in the following sections.
3. When current distribution on the electrode is unaffected by polarization or other interference factors, it is termed primary current distribution. In plating tank geometry, applying a certain voltage to two electrodes results in varying voltages at each point in the plating bath, positioned between the two electrodes.
4. Since metal electrodes are highly conductive, we can assume that the voltage at every point on the electrode surface is equal. Imaginary planes of equal potential can also be identified within the plating bath. Generally, as you approach the electrode, the equipotential plane resembles the shape of the electrode but varies in form as the distance from the electrode changes.
5. The PCB’s current density is higher where the equipotential distribution is denser and lower where it is sparser. According to electric field theory, equipotential planes and stress planes are perpendicular to each other, and the electrode itself acts as an equipotential plane. Thus, the current flowing into or out of the electrode must be perpendicular to the plane at that point.
6. The relationship between the equipotential plane of the PCB and the current distribution is crucial. Replacing the equipotential plane with a conductor or the stress plane on the equipotential surface with an insulator will not affect the electric field. However, if the equipotential surface is intersected by any substitute, the entire electric field and current distribution will change.
7. For example, using electrodes A and B, and A and C will yield the same current distribution if the BB plane coincides with the equipotential plane, thus not affecting the electric field. If electrodes A and C are slightly displaced from the center position, the equipotential lines will differ significantly from the original, as the electrode position affects the electric field and consequently alters the current distribution.
2. However, the copper plating process required for manufacturing such advanced circuit boards will encounter technical bottlenecks.
3. In recent years, the rapid advancement of the semiconductor and computer industries has made PCB production increasingly complex.
4. The complexity of a circuit board can be determined by the formula: PCB complexity index = (number of layers * number of wires between two solder joints) / (solder joint spacing in inches * wire width in mils).
5. For example, a 16-layer board with a solder joint pitch of 0.1 inches and a wire width of 5 mils, where there are three wires between the two solder joints, has a complexity index of 96.
6. Since the 1980s, the rise of surface mount technology has elevated the circuit board industry to new heights.
7. The advancement of multi-layer boards has led to a rapid increase in complexity indices, from around 20 in traditional circuit boards to 100 or higher in modern designs.
8. As technology and products evolve, encountering technical bottlenecks is inevitable.
9. Using the copper plating process as a case study, this article explores its fundamental principles and seeks solutions from three perspectives: macro, micro, and microstructure.
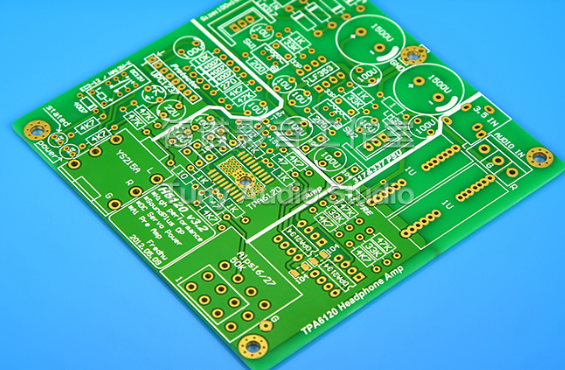
1. The macro aspect refers to the board surface of the PCB circuit board. Typically, the size of a large board is about 24″ x 18″. Achieving uniform coating thickness across the center and edges is challenging. According to Faraday’s law of electrolysis, the coating thickness is proportional to the applied current. Assuming a constant coating density, the thickness distribution mirrors the cathode current distribution.
2. Many factors affecting current distribution include the solution resistance, electrode polarization, plating geometry, and the distance between poles, as well as the magnitude of the applied current and the mass transfer rate. We will delve into these effects in the following sections.
3. When current distribution on the electrode is unaffected by polarization or other interference factors, it is termed primary current distribution. In plating tank geometry, applying a certain voltage to two electrodes results in varying voltages at each point in the plating bath, positioned between the two electrodes.
4. Since metal electrodes are highly conductive, we can assume that the voltage at every point on the electrode surface is equal. Imaginary planes of equal potential can also be identified within the plating bath. Generally, as you approach the electrode, the equipotential plane resembles the shape of the electrode but varies in form as the distance from the electrode changes.
5. The PCB’s current density is higher where the equipotential distribution is denser and lower where it is sparser. According to electric field theory, equipotential planes and stress planes are perpendicular to each other, and the electrode itself acts as an equipotential plane. Thus, the current flowing into or out of the electrode must be perpendicular to the plane at that point.
6. The relationship between the equipotential plane of the PCB and the current distribution is crucial. Replacing the equipotential plane with a conductor or the stress plane on the equipotential surface with an insulator will not affect the electric field. However, if the equipotential surface is intersected by any substitute, the entire electric field and current distribution will change.
7. For example, using electrodes A and B, and A and C will yield the same current distribution if the BB plane coincides with the equipotential plane, thus not affecting the electric field. If electrodes A and C are slightly displaced from the center position, the equipotential lines will differ significantly from the original, as the electrode position affects the electric field and consequently alters the current distribution.