As products like smartphones, tablets, and wearable devices become more compact and versatile,
the PCB industry faces the challenge of increasing layer counts while reducing thickness. The insulating layer’s thickness has dropped below the critical threshold of 50 μm, leading to declines in dimensional stability and electrical performance—particularly in signal impedance and insulation resistance. Concurrently, the density of signal traces is on the rise, with trace widths now under 40 μm. Fabricating such traces using traditional subtractive methods has become quite challenging. While additive manufacturing techniques can produce more intricate circuits, they encounter issues related to high costs and limited production scale.
This article discusses the recent challenges and advancements of ALV HDI technology in mass production, aiming to address the demands for volume, reliability, and competitive pricing in electronic packaging.
1. Overview of ALV HDI Technology

With the rise of social media, communication is increasingly conducted via smartphones and tablets. Social media has become a crucial component of any effective corporate marketing strategy. It offers a platform for engaging with both existing and potential customers, while also providing frequent feedback and fresh ideas. Consequently, the volume of data transmitted has surged in recent years and is expected to continue growing. The enhancement of functionalities alongside the miniaturization of components will be the primary driving forces behind PCB development. Semiconductor technology is advancing at an almost exponential rate, doubling every two years, a trend that is likely to persist.
2. Challenges Faced by ALV HDI PCB Manufacturing
The key production steps for the miniaturization of ALV HDI PCBs include multilayer lamination, laser drilling, imaging, etching, and electroplating. The challenge lies in optimizing these processes to achieve high-volume, robust, reliable, and cost-effective production.
1. The Evolution of Micro-Hole Laser Technology
In the mid-1990s, as component pin pitches decreased, the technical challenge became connecting high-I/O-count components with multilayer PTH PCBs. To address this, the PCB industry not only reduced the size of through holes from mechanical drilling to less than 150 mm but also developed micro-hole technologies, including photoimageable dielectric layers, plasma etching, and laser drilling. However, photoimaging requires specialized photosensitive materials, and plasma processes are ineffective on FR-4. Due to its flexibility, laser drilling has emerged as the dominant production method.
Initially, TEA CO2 and UV Nd:YAG lasers were available, but both had limitations in terms of practicality and precision. The TEA CO2 laser, with a wavelength of 10,600 nanometers, cannot effectively drill copper, operates slowly, and has a tendency for pulse misses, making its application challenging. This type of laser necessitates a conformal mask that matches or slightly exceeds the final aperture size on the copper surface. Moreover, long-wavelength laser ablation results in a carbonized layer on the PCB that must be removed using aggressive dross removal parameters.
Subsequently, some companies experimented with combining CO2 lasers and UV lasers, but this approach is primarily suitable for PCB prototypes and small production runs, proving uneconomical for larger batches.
By the mid-2000s, leading PCB manufacturers began direct drilling through copper foil, thinning the copper to between 5 mm and 12 mm and roughening the surface prior to drilling. The technical advantage of direct laser hole formation lies in eliminating the copper window etching step, significantly reducing costs. Today, this is the primary method for producing blind microvias for inter-layer connections. However, this method presents challenges due to a narrow processing window and lack of reworkability. Maintaining quality in mass production of blind microvias smaller than 100 μm is particularly challenging, as defects like overhanging copper, protruding glass fibers, and resin residue can affect subsequent desmear and electroplating processes. Thus, optimizing these micro blind holes to remove such defects is essential.
2. Electroplating and Imaging Process
The choice of PCB electroplating process is influenced by line width/spacing, insulating layer thickness, and final copper thickness. In a 0.3 mm pitch BGA design, pad diameters are 150 μm, blind holes are 75 μm, and two 30 mm/30 mm thin lines run between the pads at a 0.3 mm pitch. Producing such fine circuits with existing subtractive methods is challenging.
3. ALV HDI Technology Summary
This article highlights the critical processes involved in producing any layer interconnection PCBs and their impact on costs. When selecting a process, it is vital to ensure that the technology meets both current and future electronic packaging requirements. Challenges facing HDI PCBs include increasing functionality and size reduction, as well as the ultra-thin structures prevalent in recent consumer products. To effectively prepare materials and production methods, it is crucial to manage the supply chain efficiently, shorten prototype production cycles, and accelerate market entry for their products.
the PCB industry faces the challenge of increasing layer counts while reducing thickness. The insulating layer’s thickness has dropped below the critical threshold of 50 μm, leading to declines in dimensional stability and electrical performance—particularly in signal impedance and insulation resistance. Concurrently, the density of signal traces is on the rise, with trace widths now under 40 μm. Fabricating such traces using traditional subtractive methods has become quite challenging. While additive manufacturing techniques can produce more intricate circuits, they encounter issues related to high costs and limited production scale.
This article discusses the recent challenges and advancements of ALV HDI technology in mass production, aiming to address the demands for volume, reliability, and competitive pricing in electronic packaging.
1. Overview of ALV HDI Technology
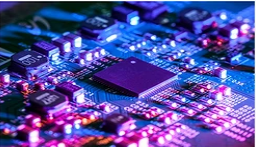
With the rise of social media, communication is increasingly conducted via smartphones and tablets. Social media has become a crucial component of any effective corporate marketing strategy. It offers a platform for engaging with both existing and potential customers, while also providing frequent feedback and fresh ideas. Consequently, the volume of data transmitted has surged in recent years and is expected to continue growing. The enhancement of functionalities alongside the miniaturization of components will be the primary driving forces behind PCB development. Semiconductor technology is advancing at an almost exponential rate, doubling every two years, a trend that is likely to persist.
2. Challenges Faced by ALV HDI PCB Manufacturing
The key production steps for the miniaturization of ALV HDI PCBs include multilayer lamination, laser drilling, imaging, etching, and electroplating. The challenge lies in optimizing these processes to achieve high-volume, robust, reliable, and cost-effective production.
1. The Evolution of Micro-Hole Laser Technology
In the mid-1990s, as component pin pitches decreased, the technical challenge became connecting high-I/O-count components with multilayer PTH PCBs. To address this, the PCB industry not only reduced the size of through holes from mechanical drilling to less than 150 mm but also developed micro-hole technologies, including photoimageable dielectric layers, plasma etching, and laser drilling. However, photoimaging requires specialized photosensitive materials, and plasma processes are ineffective on FR-4. Due to its flexibility, laser drilling has emerged as the dominant production method.
Initially, TEA CO2 and UV Nd:YAG lasers were available, but both had limitations in terms of practicality and precision. The TEA CO2 laser, with a wavelength of 10,600 nanometers, cannot effectively drill copper, operates slowly, and has a tendency for pulse misses, making its application challenging. This type of laser necessitates a conformal mask that matches or slightly exceeds the final aperture size on the copper surface. Moreover, long-wavelength laser ablation results in a carbonized layer on the PCB that must be removed using aggressive dross removal parameters.
Subsequently, some companies experimented with combining CO2 lasers and UV lasers, but this approach is primarily suitable for PCB prototypes and small production runs, proving uneconomical for larger batches.
By the mid-2000s, leading PCB manufacturers began direct drilling through copper foil, thinning the copper to between 5 mm and 12 mm and roughening the surface prior to drilling. The technical advantage of direct laser hole formation lies in eliminating the copper window etching step, significantly reducing costs. Today, this is the primary method for producing blind microvias for inter-layer connections. However, this method presents challenges due to a narrow processing window and lack of reworkability. Maintaining quality in mass production of blind microvias smaller than 100 μm is particularly challenging, as defects like overhanging copper, protruding glass fibers, and resin residue can affect subsequent desmear and electroplating processes. Thus, optimizing these micro blind holes to remove such defects is essential.
2. Electroplating and Imaging Process
The choice of PCB electroplating process is influenced by line width/spacing, insulating layer thickness, and final copper thickness. In a 0.3 mm pitch BGA design, pad diameters are 150 μm, blind holes are 75 μm, and two 30 mm/30 mm thin lines run between the pads at a 0.3 mm pitch. Producing such fine circuits with existing subtractive methods is challenging.
3. ALV HDI Technology Summary
This article highlights the critical processes involved in producing any layer interconnection PCBs and their impact on costs. When selecting a process, it is vital to ensure that the technology meets both current and future electronic packaging requirements. Challenges facing HDI PCBs include increasing functionality and size reduction, as well as the ultra-thin structures prevalent in recent consumer products. To effectively prepare materials and production methods, it is crucial to manage the supply chain efficiently, shorten prototype production cycles, and accelerate market entry for their products.