1. If both the emulator and the PCB board utilize a single power supply, should their grounds be interconnected? Ideally, using separate power supplies is preferable to minimize interference; however, many devices have specific requirements, as both the emulator and the PCB circuit board often operate with two distinct power supplies.
2. How can one verify that the PCB adheres to the design process requirements before leaving the factory? Numerous PCB manufacturers conduct a power-on network continuity test prior to the completion of processing to confirm that all connections are accurate. Additionally, an increasing number of manufacturers employ x-ray testing to identify potential failures during the etching or lamination stages. For finished boards post-patch processing, ICT testing is commonly performed, necessitating the inclusion of ICT test points during PCB design. If issues arise, specialized x-ray inspection equipment can be utilized to determine if processing has introduced faults.
3. On a 12-layer PCB featuring three power layers at 2.2V, 3.3V, and 5V, how should the ground wire be managed when all three power supplies are on one layer? Typically, the three power supplies are consolidated on the third layer to optimize signal quality. This arrangement minimizes the likelihood of signal splitting across the plane layer, as cross-segmentation significantly impacts signal integrity—a factor often overlooked by simulation software.

1. For both the power layer and the ground layer, they function similarly to high-frequency signals. In practice, it is essential to consider not only signal quality but also factors such as power plane coupling (utilizing adjacent ground planes to minimize the power plane’s AC impedance) and stacking symmetry.
2. How can crosstalk be avoided in PCB design?
A changing signal (like a step signal) travels along the transmission line from point A to point B, generating a coupled signal on transmission line CD. Once the changing signal ceases—meaning it returns to a stable DC level—the coupled signal dissipates. Consequently, crosstalk occurs only during signal transitions, and the faster the edge change (conversion rate), the greater the crosstalk produced. The electromagnetic field coupling in space can be conceptualized as a series of coupling capacitors and inductances. The crosstalk signal resulting from the coupling capacitor can be categorized into forward crosstalk and reverse crosstalk (Sc) on the victim network. Both of these signals share the same polarity. In contrast, the crosstalk generated by inductance is similarly divided into forward crosstalk and reverse crosstalk (SL), but these signals have opposite polarities. The forward and reverse crosstalk from coupled inductance and capacitance coexist and are nearly equal in magnitude. Thus, the forward crosstalk signals on the victim network tend to cancel each other out due to their opposing polarities, while the reverse crosstalk signals reinforce each other because they share the same polarity.
3. Crosstalk analysis typically includes default mode, three-state mode, and worst-case mode analysis. The default mode resembles the method used in practical crosstalk testing, where the offending network driver is activated by a flip signal while the victim network driver remains in its initial state (either high or low). The crosstalk value is then calculated, making this approach particularly effective for unidirectional signal crosstalk analysis. The three-state mode involves driving the offending network’s driver with a flip signal while setting the victim network’s tri-state terminal to a high-impedance state to measure crosstalk levels. This method is more suitable for bidirectional or complex network topologies. Worst-case analysis maintains the victim network driver in its initial state, with the simulator calculating the cumulative crosstalk from all default offending networks to each victim network. This approach typically focuses on individual critical networks due to the complexity and slow simulation speed associated with numerous combinations.
4. Is “the protection of the organization” equivalent to case protection?
Yes, the cabinet should be as tightly sealed as possible, minimizing the use of conductive materials and maximizing grounding.
5. Should multiple PCB boards in a circuit share the same ground?
In most cases, a circuit comprising multiple PCBs will require a common ground, as utilizing multiple power supplies within a single circuit is often impractical. However, under specific conditions, different power supplies may be used, resulting in reduced interference.
6. When designing a system with DSP and PLD, what ESD considerations should be addressed?
In a general system, components directly interacting with human operators require primary consideration for ESD protection, along with appropriate circuit and mechanical safeguards. The impact of ESD on the system can vary based on the environment; it is typically more pronounced in dry conditions, and sensitive systems are more susceptible. While large systems may not exhibit obvious ESD effects, careful attention to design is crucial to prevent potential issues.
7. Should I consider the ESD characteristics of the chip when selecting it?
Regardless of whether you are using a double-layer or multi-layer board, it is advisable to maximize the ground area. When selecting a chip, its ESD characteristics should be taken into account, which are usually detailed in the chip’s specifications. Different manufacturers may produce chips with varying performance levels, so comprehensive design considerations will help ensure circuit board reliability. Nonetheless, ESD issues may still arise, making organizational protection vital for ESD mitigation.
8. When creating a PCB, should the ground wire form a closed loop?
In PCB design, it is generally advisable to minimize loop areas to reduce interference. Ground wires should not be arranged in a closed loop; instead, a tree-like layout is preferred to effectively manage the ground area.
9. Designing a handheld product with an LCD and metal casing, we faced challenges passing the ICE-1000-4-2 ESD test. CONTACT could only manage 1100V, while AIR achieved 6000V. In the ESD coupling test, only 3000V was passed horizontally and 4000V vertically. With a CPU frequency of 33MHz, are there strategies to pass the ESD test?
Metallic handheld products inherently face significant ESD challenges, and LCDs can introduce additional complications. If altering the existing metal materials isn’t feasible, it is recommended to incorporate anti-static materials within the organization to enhance PCB grounding, as well as to explore methods for grounding the LCD. The specific approach will depend on the circumstances.
2. How can one verify that the PCB adheres to the design process requirements before leaving the factory? Numerous PCB manufacturers conduct a power-on network continuity test prior to the completion of processing to confirm that all connections are accurate. Additionally, an increasing number of manufacturers employ x-ray testing to identify potential failures during the etching or lamination stages. For finished boards post-patch processing, ICT testing is commonly performed, necessitating the inclusion of ICT test points during PCB design. If issues arise, specialized x-ray inspection equipment can be utilized to determine if processing has introduced faults.
3. On a 12-layer PCB featuring three power layers at 2.2V, 3.3V, and 5V, how should the ground wire be managed when all three power supplies are on one layer? Typically, the three power supplies are consolidated on the third layer to optimize signal quality. This arrangement minimizes the likelihood of signal splitting across the plane layer, as cross-segmentation significantly impacts signal integrity—a factor often overlooked by simulation software.
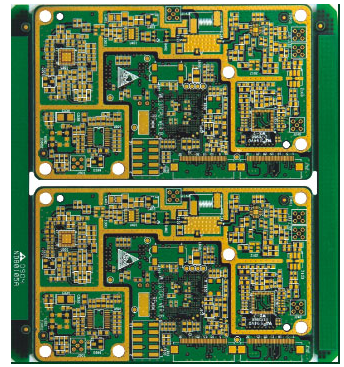
1. For both the power layer and the ground layer, they function similarly to high-frequency signals. In practice, it is essential to consider not only signal quality but also factors such as power plane coupling (utilizing adjacent ground planes to minimize the power plane’s AC impedance) and stacking symmetry.
2. How can crosstalk be avoided in PCB design?
A changing signal (like a step signal) travels along the transmission line from point A to point B, generating a coupled signal on transmission line CD. Once the changing signal ceases—meaning it returns to a stable DC level—the coupled signal dissipates. Consequently, crosstalk occurs only during signal transitions, and the faster the edge change (conversion rate), the greater the crosstalk produced. The electromagnetic field coupling in space can be conceptualized as a series of coupling capacitors and inductances. The crosstalk signal resulting from the coupling capacitor can be categorized into forward crosstalk and reverse crosstalk (Sc) on the victim network. Both of these signals share the same polarity. In contrast, the crosstalk generated by inductance is similarly divided into forward crosstalk and reverse crosstalk (SL), but these signals have opposite polarities. The forward and reverse crosstalk from coupled inductance and capacitance coexist and are nearly equal in magnitude. Thus, the forward crosstalk signals on the victim network tend to cancel each other out due to their opposing polarities, while the reverse crosstalk signals reinforce each other because they share the same polarity.
3. Crosstalk analysis typically includes default mode, three-state mode, and worst-case mode analysis. The default mode resembles the method used in practical crosstalk testing, where the offending network driver is activated by a flip signal while the victim network driver remains in its initial state (either high or low). The crosstalk value is then calculated, making this approach particularly effective for unidirectional signal crosstalk analysis. The three-state mode involves driving the offending network’s driver with a flip signal while setting the victim network’s tri-state terminal to a high-impedance state to measure crosstalk levels. This method is more suitable for bidirectional or complex network topologies. Worst-case analysis maintains the victim network driver in its initial state, with the simulator calculating the cumulative crosstalk from all default offending networks to each victim network. This approach typically focuses on individual critical networks due to the complexity and slow simulation speed associated with numerous combinations.
4. Is “the protection of the organization” equivalent to case protection?
Yes, the cabinet should be as tightly sealed as possible, minimizing the use of conductive materials and maximizing grounding.
5. Should multiple PCB boards in a circuit share the same ground?
In most cases, a circuit comprising multiple PCBs will require a common ground, as utilizing multiple power supplies within a single circuit is often impractical. However, under specific conditions, different power supplies may be used, resulting in reduced interference.
6. When designing a system with DSP and PLD, what ESD considerations should be addressed?
In a general system, components directly interacting with human operators require primary consideration for ESD protection, along with appropriate circuit and mechanical safeguards. The impact of ESD on the system can vary based on the environment; it is typically more pronounced in dry conditions, and sensitive systems are more susceptible. While large systems may not exhibit obvious ESD effects, careful attention to design is crucial to prevent potential issues.
7. Should I consider the ESD characteristics of the chip when selecting it?
Regardless of whether you are using a double-layer or multi-layer board, it is advisable to maximize the ground area. When selecting a chip, its ESD characteristics should be taken into account, which are usually detailed in the chip’s specifications. Different manufacturers may produce chips with varying performance levels, so comprehensive design considerations will help ensure circuit board reliability. Nonetheless, ESD issues may still arise, making organizational protection vital for ESD mitigation.
8. When creating a PCB, should the ground wire form a closed loop?
In PCB design, it is generally advisable to minimize loop areas to reduce interference. Ground wires should not be arranged in a closed loop; instead, a tree-like layout is preferred to effectively manage the ground area.
9. Designing a handheld product with an LCD and metal casing, we faced challenges passing the ICE-1000-4-2 ESD test. CONTACT could only manage 1100V, while AIR achieved 6000V. In the ESD coupling test, only 3000V was passed horizontally and 4000V vertically. With a CPU frequency of 33MHz, are there strategies to pass the ESD test?
Metallic handheld products inherently face significant ESD challenges, and LCDs can introduce additional complications. If altering the existing metal materials isn’t feasible, it is recommended to incorporate anti-static materials within the organization to enhance PCB grounding, as well as to explore methods for grounding the LCD. The specific approach will depend on the circumstances.