The PCB often encounters significant challenges at the beginning of the design process, particularly with regard to the substrate, layout, and routing.
Other components also require attention, and this article will address these aspects in detail.
**Noise Countermeasures on Printed Circuit Boards**
Nearly all electronic circuits rely on printed circuit boards, making the noise behavior of PCBs a critical factor in noise mitigation strategies.
The circuits on a printed circuit board can be categorized into three main types:
*Power/Ground circuit*
*Main signal circuit*
*Interface circuit*
The interface circuit plays a crucial role in connecting the PCB to external signals. Positioned strategically for noise control, this circuit serves two main purposes: preventing external noise from entering the PCB and ensuring internal noise does not radiate out. The power/ground circuit provides power to the signal and interface circuits, with the ground plane also serving as the return path for unbalanced circuits.
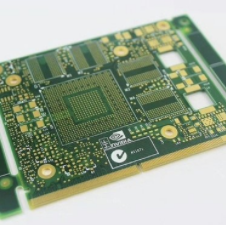
1. Originally, both the power supply and ground must maintain a stable potential. However, in reality, both the power supply and ground share a common impedance, making noise mitigation a particularly challenging aspect of circuit design.
2. From the perspective of noise control, the layout of the PCB substrate must be organized according to the type and purpose of each circuit, allowing for optimal noise mitigation strategies in the design of the printed circuit board.
3. In principle, high-noise-sensitive circuits and low-noise immunity circuits should ideally be placed on separate circuit boards. However, due to cost constraints and space limitations, it is common for these circuits to be mixed on the same board. As noted earlier, it is important to separate high-noise circuits from low-noise circuits whenever possible. In particular, signal lines carrying substantial noise should avoid long and complex routing. High-noise signal paths should be kept away from low-noise circuits, as parallel or dense routing can lead to crosstalk, increasing noise risks.
4. The way signal lines are routed depends on the configuration of the components, making component layout an essential factor in implementing these routing principles effectively.
5. On a motherboard, data transactions between substrates typically involve bus traffic. Digital circuits communicate through interface circuits at the motherboard’s edges. In addition to inter-substrate interfaces, digital circuits may also interact with external systems.
6. Analog circuits interface with external analog signals and include an A/D converter to prevent noise interference from affecting the digital circuit. As such, the A/D converter should be placed as far away from the digital interface as possible. The power supply for the analog circuit should be completely isolated from the digital circuit’s power supply. If both circuits share the same supply voltage, the noise from the analog circuit (except in the analog circuit itself) is usually minimal, allowing the analog circuit to draw power from the digital supply. In this case, a filtering device is essential to remove digital noise.
7. Regarding ground, both digital and analog circuits should connect to a single point. The ground pattern design should be irregular, creating multiple impedance paths to help separate the digital and analog units.
8. Bypass capacitors for the PCB are typically placed at the board’s power entry point to stabilize voltage and reduce noise.
9. To enhance noise control, some designs incorporate inductors and bypass capacitors to form LC filters (Figure 3). When an inductor interacts with DC, its inductance value decreases significantly. Additionally, the power supply’s inductance can generate large DC currents, making it crucial to select the correct inductor. Toroidal inductors, like those shown in Figure 4, are commonly used for this purpose. The bypass capacitors are typically arranged in a two-stage structure: one capacitor to support low frequencies and another for high frequencies.
10. The low-frequency capacitor placed at the board’s entrance typically has a capacitance based on the current demand of the board, often in the range of tens of μF, using aluminum electrolytic capacitors. High-frequency bypass capacitors are positioned near the ICs, commonly using several 0.01 μF ceramic capacitors. Ideally, a bypass capacitor should be placed as close to each IC as possible. For low-current ICs, 2–3 capacitors can be used in parallel.
11. A second bypass capacitor should be placed near each IC. If placed too far from the IC, its effectiveness may be reduced due to the inductance of the traces.
12. The use of the “Beta pattern” for power and ground planes on multilayer PCBs is highly effective. The primary advantage of the Beta pattern is its lower impedance compared to traditional linear patterns, and it also provides shielding for signal traces, making it a powerful tool for noise control in multilayer designs.
13. One of the first design principles for signal lines is to minimize their length. The layout of components plays a crucial role in determining the routing of signal traces. Most signal traces on the PCB are unbalanced, so it’s essential to consider the return paths, including ground traces, to prevent forming large area loops.
14. To prevent crosstalk, low-noise signal lines should not run parallel to high-noise signal lines. When it’s unavoidable for the ground trace between two signals to be shared, care should be taken to minimize the impact of this configuration.
15. High-impedance circuits have poorer noise immunity than low-impedance circuits. Therefore, high-impedance traces should be as short as possible, while low-impedance traces can be longer, with buffers inserted if necessary. The signal line impedance should match the characteristic impedance of the system. When high-impedance components are inserted between a driver and receiver, the trace between the component and receiver should also be kept short to avoid impedance mismatches.
16. In the past, connection issues were rare, as typical PCB designs had connection frequencies much higher than the signal frequencies (e.g., a 20 cm pattern could handle signals up to 250 MHz). The choice of ICs also depended on the signal frequency, with low-frequency ICs not exceeding their signal frequency. In this context, ICs naturally filtered high-frequency noise without causing issues.
17. However, as signal frequencies have increased in recent years, the internal signal frequency of the PCB has approached that of the connection, creating more frequent connection problems. High-frequency noise no longer only propagates through signal lines but also radiates via the signal traces, making it insufficient to rely only on filters at the receiving end. The most effective solution is to eliminate the connection altogether.
18. At high signal frequencies, delaying the signal may result in signal degradation. An alternative method is to use termination at the receiving end to eliminate the connection, though current still flows at the receiver, consuming power. For energy efficiency and other reasons, it is preferable to address noise issues at the driver-end, rather than relying solely on filters at the receiver end.
19. PCB manufacturers must master practical techniques for EMI noise mitigation in PCB design.