1. For a purely resistive load, the crosstalk current is proportional to ( frac{dI}{dt} = frac{dV}{(T_{10%-90%} cdot R)} ). In this formula, ( frac{dI}{dt} ) (rate of current change), ( dV ) (interference source voltage swing), and ( R ) (interference source load) all refer to parameters of the interference source. (If the load is capacitive, ( frac{dI}{dt} ) is inversely proportional to the square of ( T_{10%-90%} ).) From this equation, it can be observed that the low-frequency signal on the PCB does not necessarily generate less crosstalk than a high-speed signal. In other words, a 1 kHz signal is not necessarily a low-speed signal; edge characteristics must be considered in the analysis. For signals with steep edges, the harmonic content is significant, and their amplitude can be large at multiples of the fundamental frequency. Therefore, it is crucial to carefully select PCB components. Avoid choosing chips with excessively fast switching speeds, as this could not only raise costs but also exacerbate crosstalk and electromagnetic compatibility (EMC) issues.
2. Any adjacent power plane or other plane can serve as a return plane for a signal, as long as a suitable capacitor is placed at both ends of the signal to provide a low-reactive path to ground. In typical applications, the chip’s I/O power supply is shared between the transmitting and receiving ends, with decoupling capacitors of 0.01–0.1 µF commonly placed between each power supply and ground. These capacitors are also located at both ends of the signal, meaning the power plane’s return path effect is secondary only to the ground plane’s. However, when other power planes are used as return paths, the signal ends may lack a low-reactance path to ground. In such cases, the induced current in the adjacent plane will seek the nearest available capacitor to return to ground. If the “nearest capacitor” is far from the signal’s start or end, the return current must travel a long distance to complete its path. This return path is also shared with other signals, which leads to common-ground interference. Essentially, this results in crosstalk between signals.
3. For unavoidable cross-supply divisions, a high-pass filter (such as a 10-ohm resistor in series with a 680 pF capacitor) can be connected across the division. The exact values depend on the type of signal. This filter provides a high-frequency return path while isolating low-frequency crosstalk between the planes. This solution may involve adding capacitors between power planes, which might seem unconventional but is indeed effective. If the design constraints prevent this, capacitors can be routed to the ground of both planes in the division.
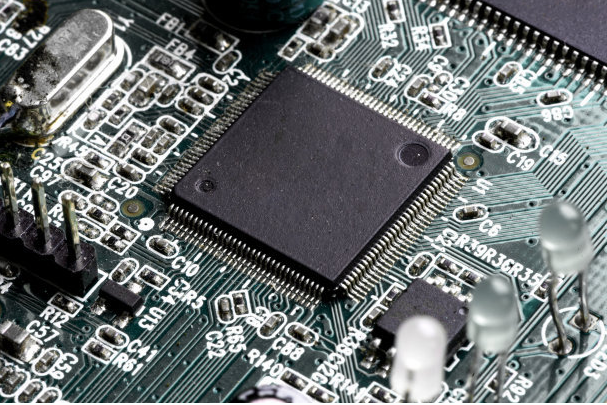
1. In cases where other planes are used for return flow, it is advisable to add a few small capacitors to the ground at both ends of the signal to provide a return path. However, this approach is often difficult to implement because the surface area near the terminals is typically occupied by the matching resistor and the chip’s decoupling capacitor.
2. Return noise is a major source of noise on the reference plane. As a result, it’s essential to study the path and range of the return current.
3. Theoretical Knowledge of PCB Current Return Paths
A circuit on the PCB is composed of traces carrying current. Normally, we only observe the signal traces on the surface, running from the driving end to the receiving end. However, the current only flows in a closed loop. The signal transmission lines are visible, while the current return path is usually hidden. Typically, return currents flow through the ground and power planes. Because there is no dedicated physical circuit for the return path, estimating and controlling it can be challenging.
4. Each trace on the PCB, along with its associated loop, forms a current path. According to electromagnetic theory, when current suddenly flows through a wire loop in a circuit, it generates an electromagnetic field in space, which can interfere with other traces. To minimize radiation, we need to first understand the basic principles of radiation and the factors that affect its intensity.
5. Differential Mode Radiation on PCB
These loops act like small antennas, radiating magnetic fields into space. By simulating the radiation generated by a small loop antenna, we can model it as a loop with current I and area S. The electric field strength measured in the far field at distance r is:
E ― Electric field (V/m)
f ― Frequency (Hz)
S ― Area (m²)
I ― Current (A)
r ― Distance (m)
θ ― Angle between the antenna and the radiation plane (°)
This formula is suitable for small loops in free space, with no reflection from nearby surfaces. In practice, however, our products are mounted on ground planes, not free space. Radiation is proportional to both the loop current and the loop area, and to the square of the frequency.
6. The return current path on a printed PCB is closely related to the frequency of the current. As per basic circuit principles, DC or low-frequency current will flow along the path of least impedance, while high-frequency current follows the path of least inductive reactance, which also involves some resistance.
7. If the effects of holes and slots created by vias on the copper plane are ignored, the path with the least impedance — which corresponds to the low-frequency current return path — forms arc-shaped paths on the ground plane. The current density along each arc is dependent on the resistivity of the area.
8. High-Frequency Current Path on the PCB Copper Plane
For transmission lines, the path with the lowest inductance — the high-frequency current return path — is directly below the signal trace on the copper-clad plane. This return path minimizes the area enclosed by the entire loop, reducing the magnetic field intensity (or the potential to radiate) of the loop antenna formed by the signal.
9. For relatively long, straight traces, the signal return current forms a band-shaped area with the signal trace at the center. The further away from the signal trace’s central axis, the lower the current density becomes.
– I ― Original signal current, in amperes (A)
– d ― Distance between the signal trace and the copper plane, in inches (in)
– h ― Vertical distance from a point on the copper plane to the signal trace, in inches (in)
– J ― Current density at this point, in amperes per inch (A/in)
10. PCB Transmission Line Return Current Density Distribution
This distribution shows the percentage of return current flowing through a band-shaped region centered around the transmission line. Assuming the width is in inches, the return current 0.035 inches away from the transmission line accounts for only 13% of the total return current. The specific distribution on one side of the transmission line is just 6.5%, with very low current density, meaning it can generally be ignored.
11. Summary:
1. When a continuous, dense, and uninterrupted copper plane is placed beneath the PCB signal traces, the noise interference caused by the return current to the copper plane is localized. By following the principles of layout and routing, such as increasing the distance between digital signal traces and analog signal traces, we can significantly reduce the impact of digital signal return currents on analog circuits.
2. The high-frequency transient return current flows back to the driver terminal through the plane (either ground or power plane) adjacent to the signal trace. The load at the driver terminal is connected across the signal trace and the plane adjacent to it.
3. The larger the surrounding area of the power and ground traces on the PCB, the greater the potential for radiation. By controlling the return path, we can minimize the loop area and therefore control the degree of radiation.
If your have any questions about PCB ,please contact me info@wellcircuits.com