The anti-interference design of a printed circuit board is closely related to the specific circuit it supports. This section will cover several common anti-interference techniques used in PCB design.
1. Power Line Design:
Based on the current requirements of the printed circuit board, increase the width of the power lines to minimize loop resistance. Additionally, ensure that the orientation of the power and ground lines aligns with the direction of data transmission, as this helps improve noise immunity.
2. Ground Wire Design Principles:

(1) The digital ground should be kept separate from the analog ground. When both logic and linear circuits are present on the same PCB, they should be physically separated as much as possible. The ground for low-frequency circuits should ideally be connected to a single point in parallel. If routing constraints make this difficult, partial series connections can be used before grounding in parallel. For high-frequency circuits, the ground should be connected at multiple points in series, with the ground traces kept as short and direct as possible. Additionally, large-area ground planes should be employed around high-frequency components whenever possible.
(2) Ground traces should be as thick as possible. If the ground traces are too narrow, the ground potential can fluctuate with changes in current, degrading the PCB’s noise immunity. Therefore, ground traces should be designed to carry at least three times the maximum current expected on the board. Where possible, ground traces should be 2-3 mm wide or more.
(3) The ground trace should form a closed loop. For PCBs that consist solely of digital circuits, the grounding network is typically arranged in a loop to enhance noise immunity.
3. **Decoupling Capacitor Configuration:**
A common practice in PCB layout design is to place appropriate decoupling capacitors at key points on the board. The general guidelines for decoupling capacitors are as follows:
(1) A 10-100 µF electrolytic capacitor should be placed at the power input terminal. If possible, a 100 µF or larger capacitor is recommended.
(2) As a rule, each integrated circuit (IC) should have a 0.01 µF ceramic capacitor. If space on the PCB is limited, a 1-10 µF tantalum capacitor can be used for every 4-8 ICs.
(3) For components with low noise immunity and large power fluctuations during shutdown, such as RAM and ROM memory devices, a decoupling capacitor should be directly connected between the chip’s power and ground pins.
(4) Capacitor leads should be kept as short as possible, especially for high-frequency bypass capacitors.
(5) When components like contactors, relays, or switches are present on the PCB and generate large spark discharges during operation, RC circuits should be used to absorb the discharge current. Typically, the resistor (R) value should be 1-2 kΩ, and the capacitor (C) value should range from 2.2 to 47 µF.
(6) CMOS inputs have very high impedance and are prone to picking up inductive noise, so unused input pins should either be grounded or connected to a positive supply when the device is in use.
1. Power Line Design:
Based on the current requirements of the printed circuit board, increase the width of the power lines to minimize loop resistance. Additionally, ensure that the orientation of the power and ground lines aligns with the direction of data transmission, as this helps improve noise immunity.
2. Ground Wire Design Principles:
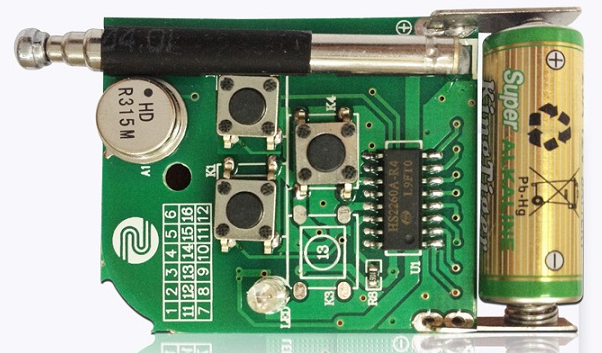
(1) The digital ground should be kept separate from the analog ground. When both logic and linear circuits are present on the same PCB, they should be physically separated as much as possible. The ground for low-frequency circuits should ideally be connected to a single point in parallel. If routing constraints make this difficult, partial series connections can be used before grounding in parallel. For high-frequency circuits, the ground should be connected at multiple points in series, with the ground traces kept as short and direct as possible. Additionally, large-area ground planes should be employed around high-frequency components whenever possible.
(2) Ground traces should be as thick as possible. If the ground traces are too narrow, the ground potential can fluctuate with changes in current, degrading the PCB’s noise immunity. Therefore, ground traces should be designed to carry at least three times the maximum current expected on the board. Where possible, ground traces should be 2-3 mm wide or more.
(3) The ground trace should form a closed loop. For PCBs that consist solely of digital circuits, the grounding network is typically arranged in a loop to enhance noise immunity.
3. **Decoupling Capacitor Configuration:**
A common practice in PCB layout design is to place appropriate decoupling capacitors at key points on the board. The general guidelines for decoupling capacitors are as follows:
(1) A 10-100 µF electrolytic capacitor should be placed at the power input terminal. If possible, a 100 µF or larger capacitor is recommended.
(2) As a rule, each integrated circuit (IC) should have a 0.01 µF ceramic capacitor. If space on the PCB is limited, a 1-10 µF tantalum capacitor can be used for every 4-8 ICs.
(3) For components with low noise immunity and large power fluctuations during shutdown, such as RAM and ROM memory devices, a decoupling capacitor should be directly connected between the chip’s power and ground pins.
(4) Capacitor leads should be kept as short as possible, especially for high-frequency bypass capacitors.
(5) When components like contactors, relays, or switches are present on the PCB and generate large spark discharges during operation, RC circuits should be used to absorb the discharge current. Typically, the resistor (R) value should be 1-2 kΩ, and the capacitor (C) value should range from 2.2 to 47 µF.
(6) CMOS inputs have very high impedance and are prone to picking up inductive noise, so unused input pins should either be grounded or connected to a positive supply when the device is in use.