1. The quality of PCB board design and wiring directly impacts the performance of the entire system. Many high-speed design theories are validated through layout. Therefore, the importance of wiring in high-speed PCB design cannot be overstated.
2. This article analyzes the rationality of encountered situations in actual wiring and proposes optimized routing strategies. The discussion focuses on three main aspects: right-angle routing, differential routing, and serpentine routing.

1. Right angle trace
Right-angle traces in PCB wiring should generally be avoided to maintain high-quality standards. They introduce impedance changes due to their sharp corners, which affect signal transmission. This impedance discontinuity can slow down signal rise times, cause signal reflections, and generate EMI. The parasitic capacitance at right angles can be estimated using the formula: ( C = 61 cdot W cdot (epsilon_r)^{1/2} / Z_0 ), where ( C ) is capacitance (pF), ( W ) is trace width (inch), ( epsilon_r ) is dielectric constant, and ( Z_0 ) is characteristic impedance. For instance, a 4 mil, 50-ohm trace (with ( epsilon_r = 4.3 )) would incur approximately 0.0101 pF due to a right angle, resulting in a rise time change estimate of 0.556 ps. Despite this, the capacitive effect is minor for general signal transmission. As trace width increases, impedance decreases, leading to signal reflection. The impedance change typically ranges from 7% to 20%, resulting in a reflection coefficient of about 0.1. This change occurs rapidly within 10 ps and is negligible for most applications below GHz frequencies. Despite concerns about EMI generation, practical tests demonstrate that right-angle traces emit EMI insignificantly compared to straight traces, often falling within instrument error margins. Thus, while right-angle routing poses minimal threats, attention to detail remains crucial, especially in high-frequency RF designs exceeding 10 GHz.
2. Differential trace
Differential signaling is increasingly favored in high-speed PCB designs due to its robustness against interference and EMI suppression. It involves sending two signals of equal magnitude but opposite phases from the driver, allowing the receiver to distinguish logic states based on voltage differences. Differential traces offer advantages over single-ended signals: strong noise immunity, effective EMI suppression through phase cancellation, and precise timing due to simultaneous switching. Engineers must ensure equal trace lengths and spacing to maintain signal polarity and impedance consistency, minimizing reflections. Ground plane continuity is crucial despite misconceptions that differential traces self-return. Proper routing practices ensure differential signals’ quality and performance, critical in LVDS and other low-amplitude signaling technologies.
3. Serpentine trace
Serpentine routing adjusts signal delay in PCB layouts but compromises signal quality and timing accuracy. Although sometimes necessary for timing alignment or to meet system requirements, serpentine traces induce coupling between parallel segments, increasing crosstalk and reducing signal integrity. To mitigate these effects, designers should increase the distance between parallel segments (at least 3 times the distance from the trace to the reference plane) to minimize mutual coupling. They should also shorten the coupling length to prevent excessive delay that could degrade signal quality. In high-speed designs, avoiding serpentine routing whenever possible is advisable, as it primarily serves timing adjustments without contributing to filtering or interference suppression.
2. This article analyzes the rationality of encountered situations in actual wiring and proposes optimized routing strategies. The discussion focuses on three main aspects: right-angle routing, differential routing, and serpentine routing.
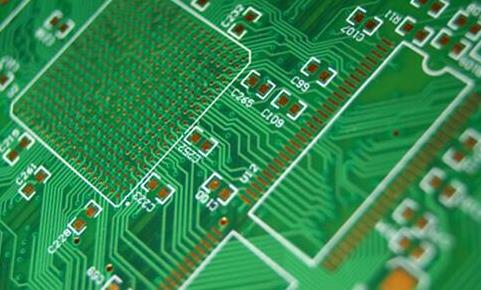
1. Right angle trace
Right-angle traces in PCB wiring should generally be avoided to maintain high-quality standards. They introduce impedance changes due to their sharp corners, which affect signal transmission. This impedance discontinuity can slow down signal rise times, cause signal reflections, and generate EMI. The parasitic capacitance at right angles can be estimated using the formula: ( C = 61 cdot W cdot (epsilon_r)^{1/2} / Z_0 ), where ( C ) is capacitance (pF), ( W ) is trace width (inch), ( epsilon_r ) is dielectric constant, and ( Z_0 ) is characteristic impedance. For instance, a 4 mil, 50-ohm trace (with ( epsilon_r = 4.3 )) would incur approximately 0.0101 pF due to a right angle, resulting in a rise time change estimate of 0.556 ps. Despite this, the capacitive effect is minor for general signal transmission. As trace width increases, impedance decreases, leading to signal reflection. The impedance change typically ranges from 7% to 20%, resulting in a reflection coefficient of about 0.1. This change occurs rapidly within 10 ps and is negligible for most applications below GHz frequencies. Despite concerns about EMI generation, practical tests demonstrate that right-angle traces emit EMI insignificantly compared to straight traces, often falling within instrument error margins. Thus, while right-angle routing poses minimal threats, attention to detail remains crucial, especially in high-frequency RF designs exceeding 10 GHz.
2. Differential trace
Differential signaling is increasingly favored in high-speed PCB designs due to its robustness against interference and EMI suppression. It involves sending two signals of equal magnitude but opposite phases from the driver, allowing the receiver to distinguish logic states based on voltage differences. Differential traces offer advantages over single-ended signals: strong noise immunity, effective EMI suppression through phase cancellation, and precise timing due to simultaneous switching. Engineers must ensure equal trace lengths and spacing to maintain signal polarity and impedance consistency, minimizing reflections. Ground plane continuity is crucial despite misconceptions that differential traces self-return. Proper routing practices ensure differential signals’ quality and performance, critical in LVDS and other low-amplitude signaling technologies.
3. Serpentine trace
Serpentine routing adjusts signal delay in PCB layouts but compromises signal quality and timing accuracy. Although sometimes necessary for timing alignment or to meet system requirements, serpentine traces induce coupling between parallel segments, increasing crosstalk and reducing signal integrity. To mitigate these effects, designers should increase the distance between parallel segments (at least 3 times the distance from the trace to the reference plane) to minimize mutual coupling. They should also shorten the coupling length to prevent excessive delay that could degrade signal quality. In high-speed designs, avoiding serpentine routing whenever possible is advisable, as it primarily serves timing adjustments without contributing to filtering or interference suppression.