**Design Principles and Anti-Interference Measures of Printed Circuit Boards**
Printed circuit boards (PCBs) serve as the foundational components for circuit elements and devices in electronic products, providing electrical connections between components and systems. As power technology advances rapidly, the density of PCBs continues to rise. PCB design significantly impacts the system’s ability to resist interference. Therefore, during the PCB design process, general design principles must be adhered to, and anti-interference requirements should be fully addressed.
**General Principles of PCB Design**
To achieve optimal performance of electronic circuits, both component placement and wiring layout play crucial roles. In designing a PCB that balances high quality and low cost, the following general principles should be considered:
1. **Layout**
The first step is to consider the size of the PCB. A PCB that is too large may result in longer traces, higher impedance, reduced noise immunity, and increased costs. On the other hand, a PCB that is too small may suffer from poor heat dissipation, and adjacent circuits could be more susceptible to interference. Once the PCB size is determined, the next step is to plan the placement of special components.

Finally, based on the functional units of the circuit, arrange all the components accordingly.
The following principles should be observed when determining the placement of special components:
(1) Minimize the connection lengths for high-frequency components, reducing their parasitic parameters and mutual electromagnetic interference.
Components sensitive to interference should not be placed too close to each other, and input and output components should be as far apart as possible.
(2) Some components or conductors may have a high potential difference, so their spacing should be increased to prevent accidental short circuits.
High-voltage components should be positioned in areas that are hard to reach during manual debugging.
(3) Components weighing more than 15g should be secured with brackets before soldering.
Heavy components that generate heat should not be installed directly on the PCB, but instead mounted on the machine’s bottom, considering heat dissipation requirements.
Heating elements should be kept away from other components that also generate heat.
(4) The placement of adjustable components like potentiometers, variable inductors, variable capacitors, and microswitches should account for the mechanical design of the entire device.
If adjustments need to be made inside the device, they should be positioned on the PCB for easy access.
If adjustments are made externally, the components should align with the adjustment knobs on the chassis panel.
(5) The areas occupied by the positioning holes for the print wrench and fixing brackets should be set aside, in line with the functional unit arrangement of the circuit.
When arranging the components of the circuit, the following principles must be adhered to:
(1) Position each functional circuit unit according to the signal flow, ensuring that the layout supports the easy flow of signals and maintains a consistent direction.
(2) Treat the core component of each functional unit as the center, with surrounding components arranged evenly, neatly, and compactly on the PCB.
Minimize and shorten the traces and connections between components.
(3) For high-frequency circuits, consider the parasitic parameters between components.
In general, parallel connections should be used wherever possible. This not only improves aesthetics but also makes installation and soldering easier, facilitating mass production.
(4) Components should be placed at least 2mm from the edges of the PCB, with the preferred shape of the board being rectangular, with an aspect ratio of 3:2 to 4:3.
For boards larger than 200x150mm, mechanical strength should also be considered.
**2. Wiring**
The principles for wiring are as follows:
(1) Avoid running input and output wires in adjacent parallel paths.
Ideally, ground wires should be placed between signal lines to prevent feedback coupling.
(2) The minimum width of printed traces depends on the bond strength between the trace and the insulating substrate, as well as the current they carry.
For a 0.05mm thick copper foil and traces with widths between 1-15mm, a current of 2A will cause no more than a 3°C temperature rise. Therefore, a trace width of 1.5mm is sufficient.
For integrated and especially digital circuits, trace widths typically range from 0.02mm to 0.3mm. However, whenever possible, wider traces should be used, especially for power and ground lines.
The minimum spacing between wires, in the worst case, is determined by the insulation resistance and breakdown voltage.
For digital circuits, the spacing can be as small as 5~8mm, provided the manufacturing process allows.
(3) Printed traces at corners should generally be arc-shaped. Right angles or sharp bends in high-frequency circuits can degrade electrical performance.
Also, avoid using large areas of copper foil as they may swell and detach due to prolonged heating.
When large areas of copper foil are unavoidable, a grating pattern should be used to help remove volatile gases from the adhesive layer.
**3. Soldering Pad Hole Sizes**
The center hole of a soldering pad should be slightly larger than the lead diameter of the component.
If the hole is too large, it may lead to a cold joint.
The outer diameter of the pad should generally not be less than (d + 1.2)mm, where d is the diameter of the lead.
For high-density digital circuits, the ideal minimum pad diameter is (d + 1.0)mm.
**PCB and Circuit Anti-Interference Measures**
The anti-interference design of a printed circuit board is closely related to the specific circuit. Here, we will discuss several commonly used anti-interference measures.
1. **Power Line Design**
The power line should be as wide as possible to reduce loop resistance, based on the current requirements of the PCB.
Additionally, ensure that the power, ground, and signal lines are aligned in the same direction to improve noise immunity.
2. **Ground Wire Design**
The following principles should be followed in designing the ground line:
(1) Separate the digital ground from the analog ground.
If both logic and analog circuits are present, they should be kept as separate as possible. Low-frequency circuits should ideally use a single grounding point, but if this is difficult, parts of the ground can be connected and then grounded.
High-frequency circuits should have multiple grounding points, and the ground lines should be kept short and thick.
Where possible, large-area grating should be used to shield high-frequency components.
(2) The ground wire should be as thick as possible. Thin ground traces cause voltage fluctuations due to current flow, which reduces noise immunity.
Ground traces should be thick enough to carry three times the maximum current expected. If possible, the ground trace should be at least 2-3mm wide.
(3) A closed-loop ground configuration should be used whenever possible.
For PCBs composed of only digital circuits, creating a ground loop can significantly improve noise resistance.
3. **Decoupling Capacitor Configuration**
A common practice in PCB design is to place decoupling capacitors at key locations throughout the circuit.
General guidelines for decoupling capacitors include:
(1) A 10~100µF electrolytic capacitor should be placed at the power input, and ideally, this should exceed 100µF.
(2) A 0.01µF ceramic capacitor should be placed for each IC chip. If space is limited, 1~10pF capacitors can be placed for every 4~8 chips.
(3) For components with poor noise immunity or large power fluctuations (e.g., RAM and ROM), the decoupling capacitor should be directly connected between the power line and the chip’s ground.
(4) Capacitor leads should be kept as short as possible, especially for high-frequency bypass capacitors.
Additionally, note the following:
(1) When components like relays, switches, or buttons are on the PCB, they may generate large spark discharges during operation. These discharges must be absorbed using an RC circuit (R = 1~2kΩ, C = 2.2~47µF).
(2) CMOS components have high input impedance and are more susceptible to noise, so grounding them or inserting a pull-down resistor at the unused end is highly recommended.
Printed circuit boards (PCBs) serve as the foundational components for circuit elements and devices in electronic products, providing electrical connections between components and systems. As power technology advances rapidly, the density of PCBs continues to rise. PCB design significantly impacts the system’s ability to resist interference. Therefore, during the PCB design process, general design principles must be adhered to, and anti-interference requirements should be fully addressed.
**General Principles of PCB Design**
To achieve optimal performance of electronic circuits, both component placement and wiring layout play crucial roles. In designing a PCB that balances high quality and low cost, the following general principles should be considered:
1. **Layout**
The first step is to consider the size of the PCB. A PCB that is too large may result in longer traces, higher impedance, reduced noise immunity, and increased costs. On the other hand, a PCB that is too small may suffer from poor heat dissipation, and adjacent circuits could be more susceptible to interference. Once the PCB size is determined, the next step is to plan the placement of special components.
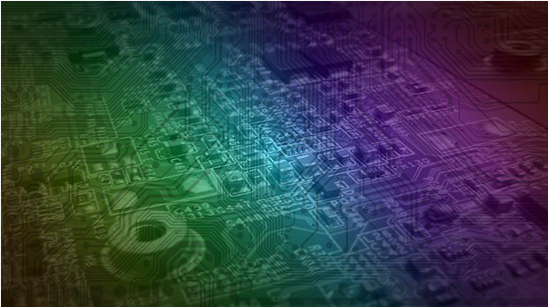
Finally, based on the functional units of the circuit, arrange all the components accordingly.
The following principles should be observed when determining the placement of special components:
(1) Minimize the connection lengths for high-frequency components, reducing their parasitic parameters and mutual electromagnetic interference.
Components sensitive to interference should not be placed too close to each other, and input and output components should be as far apart as possible.
(2) Some components or conductors may have a high potential difference, so their spacing should be increased to prevent accidental short circuits.
High-voltage components should be positioned in areas that are hard to reach during manual debugging.
(3) Components weighing more than 15g should be secured with brackets before soldering.
Heavy components that generate heat should not be installed directly on the PCB, but instead mounted on the machine’s bottom, considering heat dissipation requirements.
Heating elements should be kept away from other components that also generate heat.
(4) The placement of adjustable components like potentiometers, variable inductors, variable capacitors, and microswitches should account for the mechanical design of the entire device.
If adjustments need to be made inside the device, they should be positioned on the PCB for easy access.
If adjustments are made externally, the components should align with the adjustment knobs on the chassis panel.
(5) The areas occupied by the positioning holes for the print wrench and fixing brackets should be set aside, in line with the functional unit arrangement of the circuit.
When arranging the components of the circuit, the following principles must be adhered to:
(1) Position each functional circuit unit according to the signal flow, ensuring that the layout supports the easy flow of signals and maintains a consistent direction.
(2) Treat the core component of each functional unit as the center, with surrounding components arranged evenly, neatly, and compactly on the PCB.
Minimize and shorten the traces and connections between components.
(3) For high-frequency circuits, consider the parasitic parameters between components.
In general, parallel connections should be used wherever possible. This not only improves aesthetics but also makes installation and soldering easier, facilitating mass production.
(4) Components should be placed at least 2mm from the edges of the PCB, with the preferred shape of the board being rectangular, with an aspect ratio of 3:2 to 4:3.
For boards larger than 200x150mm, mechanical strength should also be considered.
**2. Wiring**
The principles for wiring are as follows:
(1) Avoid running input and output wires in adjacent parallel paths.
Ideally, ground wires should be placed between signal lines to prevent feedback coupling.
(2) The minimum width of printed traces depends on the bond strength between the trace and the insulating substrate, as well as the current they carry.
For a 0.05mm thick copper foil and traces with widths between 1-15mm, a current of 2A will cause no more than a 3°C temperature rise. Therefore, a trace width of 1.5mm is sufficient.
For integrated and especially digital circuits, trace widths typically range from 0.02mm to 0.3mm. However, whenever possible, wider traces should be used, especially for power and ground lines.
The minimum spacing between wires, in the worst case, is determined by the insulation resistance and breakdown voltage.
For digital circuits, the spacing can be as small as 5~8mm, provided the manufacturing process allows.
(3) Printed traces at corners should generally be arc-shaped. Right angles or sharp bends in high-frequency circuits can degrade electrical performance.
Also, avoid using large areas of copper foil as they may swell and detach due to prolonged heating.
When large areas of copper foil are unavoidable, a grating pattern should be used to help remove volatile gases from the adhesive layer.
**3. Soldering Pad Hole Sizes**
The center hole of a soldering pad should be slightly larger than the lead diameter of the component.
If the hole is too large, it may lead to a cold joint.
The outer diameter of the pad should generally not be less than (d + 1.2)mm, where d is the diameter of the lead.
For high-density digital circuits, the ideal minimum pad diameter is (d + 1.0)mm.
**PCB and Circuit Anti-Interference Measures**
The anti-interference design of a printed circuit board is closely related to the specific circuit. Here, we will discuss several commonly used anti-interference measures.
1. **Power Line Design**
The power line should be as wide as possible to reduce loop resistance, based on the current requirements of the PCB.
Additionally, ensure that the power, ground, and signal lines are aligned in the same direction to improve noise immunity.
2. **Ground Wire Design**
The following principles should be followed in designing the ground line:
(1) Separate the digital ground from the analog ground.
If both logic and analog circuits are present, they should be kept as separate as possible. Low-frequency circuits should ideally use a single grounding point, but if this is difficult, parts of the ground can be connected and then grounded.
High-frequency circuits should have multiple grounding points, and the ground lines should be kept short and thick.
Where possible, large-area grating should be used to shield high-frequency components.
(2) The ground wire should be as thick as possible. Thin ground traces cause voltage fluctuations due to current flow, which reduces noise immunity.
Ground traces should be thick enough to carry three times the maximum current expected. If possible, the ground trace should be at least 2-3mm wide.
(3) A closed-loop ground configuration should be used whenever possible.
For PCBs composed of only digital circuits, creating a ground loop can significantly improve noise resistance.
3. **Decoupling Capacitor Configuration**
A common practice in PCB design is to place decoupling capacitors at key locations throughout the circuit.
General guidelines for decoupling capacitors include:
(1) A 10~100µF electrolytic capacitor should be placed at the power input, and ideally, this should exceed 100µF.
(2) A 0.01µF ceramic capacitor should be placed for each IC chip. If space is limited, 1~10pF capacitors can be placed for every 4~8 chips.
(3) For components with poor noise immunity or large power fluctuations (e.g., RAM and ROM), the decoupling capacitor should be directly connected between the power line and the chip’s ground.
(4) Capacitor leads should be kept as short as possible, especially for high-frequency bypass capacitors.
Additionally, note the following:
(1) When components like relays, switches, or buttons are on the PCB, they may generate large spark discharges during operation. These discharges must be absorbed using an RC circuit (R = 1~2kΩ, C = 2.2~47µF).
(2) CMOS components have high input impedance and are more susceptible to noise, so grounding them or inserting a pull-down resistor at the unused end is highly recommended.